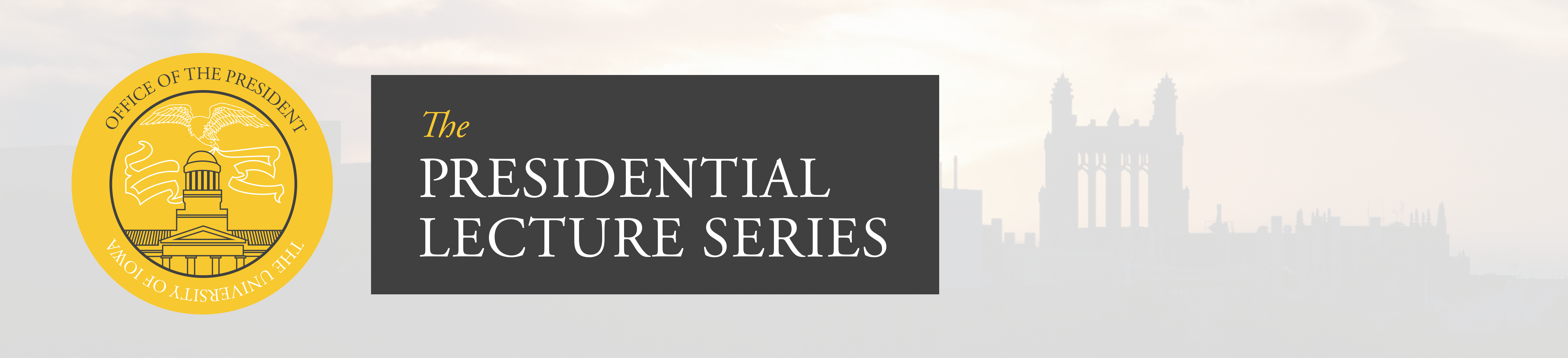
16th Annual Presidential Lecture Series
https://doi.org/10.17077/yv51-g50m
Small Comets and Our Origins:
The Ecstasy and Agony of the Scientific Debate
Carver/James A. Van Allen Professor of Physics
Department of Physics and Astronomy
The University of Iowa
It is a shocking notion. Radiation-blasted, black-jacketed snowballs the size of houses swarming out of the cosmic void every day, plowing into Earth’s atmosphere and pancaking explosively into clouds of vapor that fall as gentle rains.
Over billions of years these invaders might have filled Earth’s oceans and provided the seeds of life.
President Coleman, colleagues, and friends, I am honored by your presence at my lecture.
The beginning of the debate
This is a narrative of an intense debate about the findings of a startlingly large influx of small comets into our atmosphere. This debate is now thirteen years old and is characterized by numerous emotional and intellectual statements to be found in both the scientific literature and the popular press. If the small comets exist, then fundamentally new insight into such questions as the origins of our oceans, of the influx of organic material from space, and, indeed, of our own origins is gained. The scientific debate is very much alive.
The saga began with the launch of three University of Iowa cameras on board the Dynamics Explorer-1 spacecraft in 1981. The scientific objectives of these cameras were to provide the first comprehensive series of global images of one of Earth’s natural marvels, the crowns of auroral lights to be found encircling its two poles. The acquisition of these images of the aurora was successful beyond even our considerable expectations, and they are widely used in scientific articles, textbooks, and the popular press.
However, there was an important feature which was a puzzle from the time of the receipt of the initial images in space. This puzzle was the quite frequent appearance of dark “spots” in the images which inevitably drew the attention of scientists who were attending our talks on the auroral lights. Our response to these early queries as to the cause of these dark “spots” was that they were due either to an artifact of the camera or the way the images were transmitted to Earth from the spacecraft. The task to explain these dark spots as noise was assigned to John Sigwarth, a very promising graduate student of mine. After several years of intensive studies of the cameras and their images we failed to show that the dark spots in the images were due to noise in the camera. The behavior of these spots as functions of spacecraft position and time, as well as their correlation with observations of radar meteors, forced us to the unsettling conclusion that the spots were real.
As we shall see, the implications of the reality of the dark spots in the images were not accommodated by the standard acceptance criteria known as current wisdom. There were two choices available to us: put the results into our desk drawers and lead a relatively peaceful life, or publish the results and suffer the criticism, and the sometimes extreme animosity of colleagues and previous friends. There was only one choice with integrity in this matter; the work had to be submitted for publication.
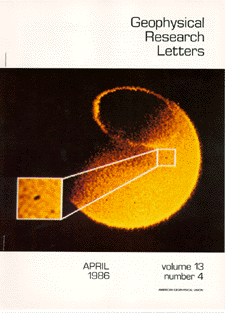
The two papers on the dark spots were accepted within a remarkably short review period of 48 hours by the editor, Alexander Dessler, of one of the leading scientific journals in geophysics, Geophysical Research Letters. The papers were accepted over the strongly felt objections of the two reviewers, one of whom stated that “if the contents of the papers are correct then half the physical science books in our libraries will have to be destroyed.” The papers were featured on the cover of this journal, reproduced in Figure 1. A global picture of Earth taken at extreme ultraviolet wavelengths beyond the capability of our eyes is shown. In fact these wavelengths are dangerous to our eyesight and are shielded from us by our atmosphere. The lights in Figure 1 are located 200 to 300 miles above Earth’s surface. The general glow which looks like the illumination of a ball by a light bulb is indeed the atmospheric glow which is due to the illumination of our upper atmosphere by sunlight. The second feature of note in Figure 1 is the “ring” of auroral lights in the upper portion of the image. This remarkable ring of auroral lights encircles the North Pole and was the primary objective of the camera. The catalyst of the great debate is the appearance of the dark spots in the atmosphere, one of which is shown in the inset.
Given the reality of the dark spots, which soon became known as “atmospheric holes” because of their appearance in the images, there is only one explanation which has endured over all these years to present. That is, the holes are due to the shadowing of the atmospheric light by an object above the atmosphere. This object simply cannot be a stony or iron meteor because the holes are very large, tens of miles in diameter. A rock of this size would provide a disastrous impact on the Earth’s surface. As it turns out, water vapor is very good at absorbing the atmospheric light and thus appearing as an atmospheric hole in the images taken by the spacecraft camera. The only other step in the interpretation is to note that a cloud of water vapor will have only a brief existence in interplanetary space so that it must be delivered to Earth as a small comet filled with water snow which is disrupted and expands as it impacts into our atmosphere.
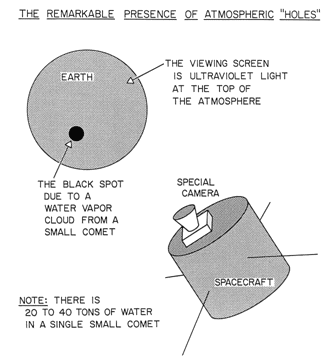
A diagram of the camera as it views an atmospheric hole is shown in Figure 2. In principle the detection of the atmospheric hole above our upper atmosphere is much like observing a fly walking across a television screen. Of course, Earth is much larger, as is the size of the atmospheric holes. The amount of cometary water vapor which is necessary to provide the atmospheric hole is in the range of 20 to 40 tons. The history of the impact of a small comet with this amount of water snow as it arrives from interplanetary space is shown in Figure 3. The fragile comet is disrupted at altitudes of roughly 800 miles just above our atmosphere. Sunlight is a very efficient energy source for converting the cometary snow into a water cloud. The small comet approaches the Earth at speeds of approximately 35,000 miles per hour. The diameter of a small comet before disruption is typically tens of feet, typical of a small house, but the expansion of the water cloud after its release from the disrupted small comet is rapid. This rapid expansion provides a cometary water cloud of tens of miles in size, and the impact with the atmosphere is relatively benign. The water cloud is then slowed and mixed with the upper atmosphere. This cometary water cloud is subsequently deposited at Earth’s surface as a gentle “cosmic rain.”
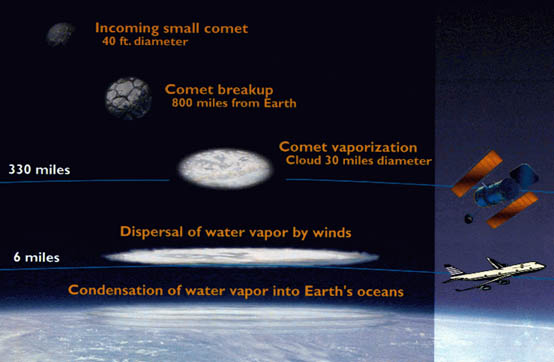
This is nothing greatly objectionable about the presence of small comets or about the above impact scenario. The objections are directed toward the numbers, the numbers of such objects required to explain the yearly rates of atmospheric holes. A yearly rate of 1 to 10 events in our atmosphere would be generally accepted by the scientific community, 10 to 100 events might be forgiven, but a thousand such impacts raise considerable outcries on the basis of “current wisdom.” The proposed rate of 10 million small comets impacting our atmosphere each year was generally greeted with scorn and ridicule. Remarkably, at this rate over the age of Earth, the small comets can provide enough water to fill our oceans.
Thousands of scientists in many disciplines of physical sciences such as geology, oceanography, and planetary studies found the proposed existence of small comets to be simply folly. Indeed it is fair to say that the only scientists to accept their existence were the three authors of the original two papers: research scientist John Craven, who was in charge of the camera design, Sigwarth, and myself. The criticism and emotional reactions began immediately with their publication. Noting that the publication date was April 1, 1986, reporters from newspapers, including the New York Times, quickly called to confirm that the content of the papers was simply an “April Fool’s joke.” The attitude of the scientific community was epitomized by the following consensus opinion.
One has as much chance to see a small comet’s shadow in Earth’s atmosphere as to be able to show that a pig flies by seeing its shadow in moonlight.
This is a clever retort but one must give credit to the source of inspiration, a Weekly World News report from Jakarta, Indonesia, reproduced in Figure 4: “Farmers shot up an entire herd of flying pigs as the migrating porkers flew over their rice paddies.”
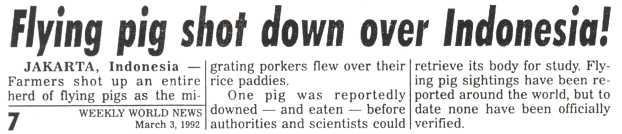
In the face of a multitude of such criticisms it would have been advantageous to our professional careers if we could have declared that the atmospheric holes were just due to camera noise in the images from the Dynamics Explorer spacecraft. But this was not possible for four major reasons. Firstly most of the holes moved from dusk to dawn across the dayside atmosphere. The camera would have no internal knowledge of such favoritism. Secondly and thirdly, the atmospheric holes favored the late morning hours and also became larger as the spacecraft moved to lower altitudes, respectively. Again, the camera had no knowledge of what part of the atmosphere was being viewed or of the spacecraft position. And finally the frequency of atmospheric holes was correlated with the seasonal variations of meteors as viewed from a ground-based radar station. No retreat from the reality of atmospheric holes was possible.
The new cameras
Images from cameras on other spacecraft which could confirm the existence of atmospheric holes were not available until the launch of the Polar spacecraft on February 24, 1996. The reasons for this were several: the images must be taken in the special ultraviolet window, provide a view of a large section of the daylit atmosphere, and have sufficient resolution to detect an atmospheric hole. The intervening interval of ten years since the report of images from Dynamics Explorer- 1 was a period of relatively quiescent activity during which the scientific community became further convinced that the atmospheric holes in the images were simply due to camera noise. In this quiet atmosphere another set of auroral cameras was being constructed at the University of Iowa for the Polar spacecraft. The capabilities for the detection of atmospheric holes, and of the small comets, were also objectives of these cameras. These cameras were the most sophisticated scientific instruments ever built at the University of Iowa. If atmospheric holes and the small comets existed, the resolutions and capabilities of these cameras would leave no doubt.
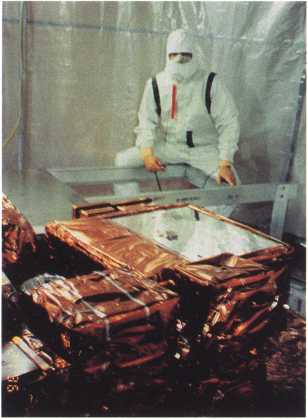
The scientist in charge of the design and construction of the sophisticated Polar cameras was John Sigwarth, who now was a senior research scientist. Of the original three researchers in the efforts with the cameras on board Dynamics Explorer-1, John Craven had departed to accept a faculty position at the University of Alaska at Fairbanks. The new Polar cameras shown in Figure 5, were large and heavily instrumented with onboard computers. The person in the “space suit” is indeed John Sigwarth and he is sitting at the top of the 100-foot tall gantry which allows him to view the cameras. The cameras are encased within the largest rectangular box in front of him. The rest of the spacecraft is hidden below the cameras. The spacecraft and the cameras would be soon launched into a high-altitude orbit above the Northern Hemisphere. It would have a superb view of both the auroral lights and of the sunlit atmosphere.
These new cameras were capable of greatly extending the previous observations with Dynamics Explorer-1 in three important, independent ways. First of all the atmospheric holes could be observed with much greater spatial resolution, which provides confirmation beyond any reasonable claims of “camera noise.” Secondly, the sky in the vicinity of our planet could be monitored for bright trails due to the glow of oxygen from the disruption of some of the comets at very high altitudes of thousands of miles. Thirdly, the new cameras would allow optical detection of the fragments of water molecules as the cometary clouds plummeted into the atmosphere.
The Polar spacecraft and its cameras were successfully launched and the complex process of turning on these sophisticated cameras proceeded during the month of March 1996. John Sigwarth was at the control center at NASA’s Goddard Space Flight Center in Greenbelt, Maryland during this critical period. I will always remember a telephone conversation with John during which a lengthy discussion of camera voltages, currents, and temperatures occurred. At the end of this conversation, John quietly said “Oh, yes, by the way, the atmospheric holes are clearly present in the images.”
Although John and I knew that the above three confirmations were successful by late Fall of 1996, it was clear to us that we needed some “quiet time” to achieve a careful, thorough analysis. Our silence during this period of over a year after launch was greeted by most scientists with relief that the atmospheric holes and small comets must not exist. When the results were finally reported at a NASA-supported press conference at the American Geophysical Union meeting during May 1997, there was great turmoil and confusion in the scientific community and the announcements raced like a wildfire through the press.
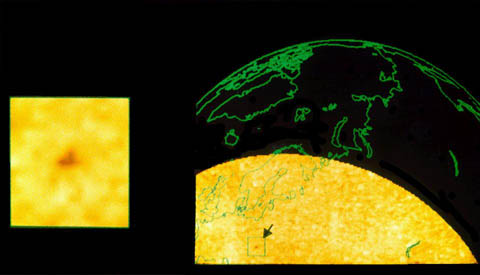
The confirmation of the existence of atmospheric holes with the Polar cameras is demonstrated by the ultraviolet image shown in Figure 6. This atmospheric hole is positioned above Poland and was recorded on April 6, 1996. It is remarkable that the rate at which these atmospheric holes occur in the atmosphere is very similar to that previously observed with Dynamics Explorer-1, even though the cameras were greatly different.
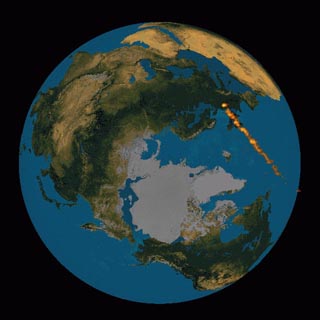
The second independent confirmation was also provided by the camera for ultraviolet wavelengths. A few of the small comets were disrupted at much higher altitudes than for the vast majority of comets impacting the atmosphere. For those which were bursting at the higher altitudes, trails of bright glows from atomic oxygen were clearly observed. An example of such a trail is shown in Figure 7. This picture was taken on September 26, 1996. The bright trail of the gases from the disrupted comets has been superimposed upon a map of Earth generated by a commercial computer program in order to provide perspective as to the view seen by the Polar camera. The trail moves across the Atlantic Ocean and ends above Eastern Germany. Such photographs never had been taken by any previous spacecraft. Note that the images in both Figures 6 and 7 show atmospheric impacts at locations other than the North American continent. Because of the emotional reactions of a group of scientists in the United States to the amazing set of new images, we had decided that it was best to show impacts in other locations for the first press releases in order to ameliorate the situation. Indeed cometary trails above the Northern Hemisphere are observed. An example of a trail captured in a photograph on September 28, 1996 above San Diego, California is shown in Figure 8. Such pictures provide undeniable evidence that a new class of objects has been discovered in the vicinity of Earth.
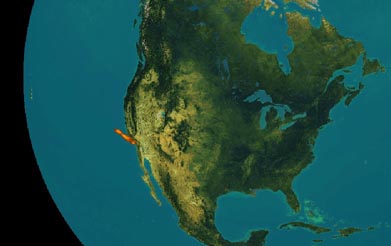
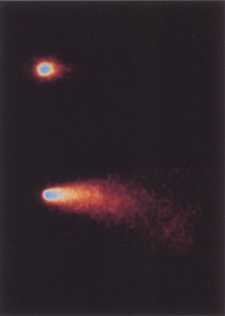
Finally, as a third independent verification of the existence of the small comets, another of the three cameras was used to search for the cometary water impacts into the atmosphere. These pictures were taken in light that was nearer to the range of our eyesight but still a little too far into the violet to see. However, this was a wavelength which is used frequently to monitor the well-known large comets. This light is emitted as the Sun illuminates the fragments of cometary water vapor, specifically the fragment produced when a hydrogen atom is stripped from a water molecule. In order to calibrate our cameras on the Polar spacecraft we pointed them toward Comet Hale-Bopp as it approached the Sun. This was very exciting and instructive for us. Images in two different colors of light are shown in Figure 9. The top picture shows this large comet as seen in the light from the fragments of water vapor as noted above. The bottom photograph is taken in the light emitted by sodium and also light scattered from cometary dust. The sodium is to be found in the remarkable thin trail at the top and the rest is due to dust. Thus the Polar cameras were shown to be very capable of detecting cometary lights.
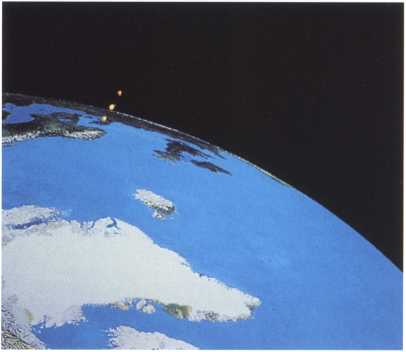
But there was a surprise awaiting us when we turned the cameras to view the small comets which were impacting the Earth’s upper atmosphere. The light from the water fragments was dramatically seen in the sequences for each comet, one sequence of which is shown in Figure 10. The surprise was the absence of sodium and dust in these water clouds of the disrupted small comets. This provided the explanation for one puzzling aspect of the small comets, the absence of very bright trails during the atmospheric impacts. Sodium and dust provide bright impact glows, but water and its fragments are much dimmer. Thus the composition of the small comets is significantly different than that of the well-known large comets. It was rewarding to compute the amount of water vapor in the small comets from measurements of the brightness of the light emitted by the water fragments. The mass of water in a single comet was about 20 to 40 tons, and confirmed the result obtained independently from observations of atmospheric holes. Complete closure was obtained when the measured frequency of water trails such as that shown in Figure 10 was found to be very similar to the occurrence rate of atmospheric holes such as that displayed in Figure 6.
The opinions of the scientific community greatly changed after the announcement of the Polar results in May 1997. A majority of the scientists offered congratulations on the confirmation of the earlier Dynamics Explorer results. The public was very supportive of these findings which promised to provide new insight into our origins. But a minority of scientists, very vocal and organized, fought against the breach in dogmas offered by the small comet findings. There were presentations of results and opinions which drew more than a thousand spectators at the national meetings of the American Geophysical Union. The atmosphere was extremely combative and the presentations were then based upon emotions rather than sound scientific reasoning. Of course, it was immensely entertaining for those who were merely spectators. A good characterization of these heated encounters was offered by science writer Patrick Huyghe, who has closely collaborated with me on numerous occasions in communicating with the public and the scientific community, in the [Frank Cotham New Yorker] cartoon shown in Figure 11 [Not shown. This cartoon shows two men crouched behind a speaker’s table. Bullets are flying over them in the air. While one man fires back with a machine gun, the other says: “This symposium has gotten completely out of hand!”].
Contributions to our knowledge of Earth, and thus of our origins
An argument used to deny the existence of the small comets relies upon scientific dogmas which explain all facets of our planet and our origins with absolutely no need for a role for the small comets. But over the past ten years or so our advances in technology have revealed exciting new results which are beyond the reach of the dogmas. The discovery of the small comets is one such example of the advancement of our scientific frontiers. We summarize here several of the important implications of this swarm of small, dark comets which are present in the vicinity of our planet.
The origins of our oceans always have been a fascinating mystery. In his classic paper of 1951 William Rubey noted that an inventory of Earth’s water gave the following results:
Earth’s Water Inventory (in units of 100 million million tons) | ||
---|---|---|
In present hydrosphere, atmosphere and biospere | 14,600 | |
Buried in ancient rocks | 2,100 | |
Total | 16,700 | |
This is to be compared with the supply by weathering of rocks | 130 | |
Then the water unaccounted for is a remarkable | 16,600 |
Rubey was puzzled by the large amount of water that was unaccounted for. Even at the time of writing his seminal paper he queried astronomers as to whether it was possible that this water was being supplied by an infall of objects from interplanetary space. The responses of astronomers were negative. The mystery was not resolved.
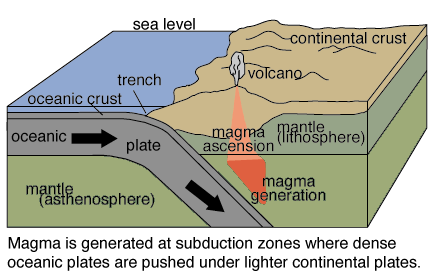
But our knowledge of the geological dynamics of Earth is currently expanding at a rapid rate. Large amounts of water are now believed to be lost as subduction of continental plates carries oceanic water under the surface. This scenario is depicted in Figure 12. This water was thought to be recycled to the surface by outflow of gases from volcanic activity. This was a natural suggestion in consideration of the dramatic activity of volcanoes. A picture of such a volcano is shown in Figure 13. This is Paricutin, which was born in a Mexican corn field in 1943. In a recent classic paper of 1999 David Deming reports on the amount of water returned to the Earth’s surface by volcanic activity and finds that:
The losses of surface water due to subduction into the mantle are greater by factors of 7 to 20 than the supply given by volcanic activity.
The rate of a cosmic influx of water to compensate for the water loss to the mantle is similar to that derived by Frank and Sigwarth [1993] from observations of small comets.
The significance of the small comets is obvious: our Earth would be dry and barren without an extraterrestrial influx of water.
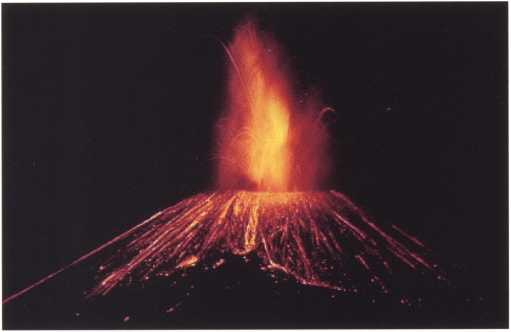
But a further conclusion in Deming’s paper is unsettling:
Life on Earth may be balanced precariously between cosmic processes which deliver an intermittent stream of life sustaining volatiles from the outer solar system or beyond, and biological and tectonic processes which remove these same volatiles from the atmosphere by sequestering water and carbon in the crust and mantle.
One of the arguments used against the existence of the small comets was the extreme dryness of our atmosphere above altitudes of 20 to 30 miles. The claim was that, if there was such a large flux of cometary water clouds entering our atmosphere, then surely there must be some evidence of the water vapor at high altitudes. The lack of such water vapor was cast into cartoon form as “falling chickens” as shown in Figure 14 [Not shown. The Ken Brown cartoon spoofs the many impacts of small comets into our atmosphere. Captioned “The effects of rubber chickens on the atmosphere,” it shows a scientist recording in a pad the falls of rubber chickens all around him].
However, surprises concerning the amount of water vapor in our high atmosphere were revealed with instruments flown on the Space Shuttle and on the unmanned Upper Atmospheric Research Satellite. These measurements of unexpected quantities of water vapor at altitudes in our mesosphere in the range of 40 miles or so are depicted in Figure 15.
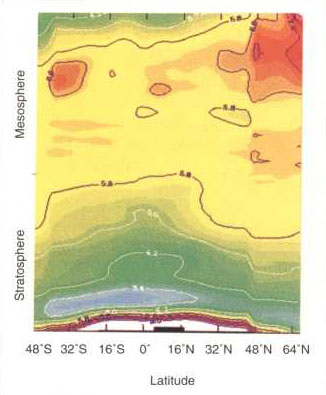
James M. Russell III, HALOE’s lead scientist and an atmospheric physicist at Hampton (Va.) University.
—R. Monastersky
Figure 15. The recent discovery of an unexplained excess of water vapor at high altitudes in our atmosphere.
Although most of the cometary water will not be stopped in its rapid downward motion until it reaches the stratosphere, a small amount of water vapor is expected to be left at the high altitudes. The findings of such water vapor, a measurement which is a significant technical achievement, quieted many of the objections which were based upon the “very dry upper atmosphere.” A further concern now arises if the upper atmosphere is bathed with a gentle cosmic rain. That concern is the existence of high-altitude ice clouds at about 55 miles. A photograph of these clouds which are found at polar latitudes is shown in Figure 16. If these clouds are due to the presence of cometary water vapor and their fluxes were to increase, then these clouds could advance toward lower latitudes. An ice age would ensue.
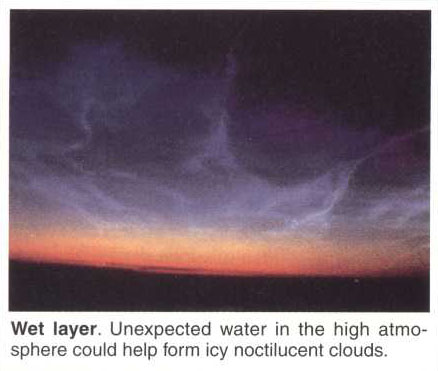
Even though the small comets were a frequent topic for the press and were quite popular with the public several icons of the American Geophysical Union were successful in preventing any article concerning them in the union’s newsletter EOS. This censure continued for over ten years until the confirmations with the Polar cameras.
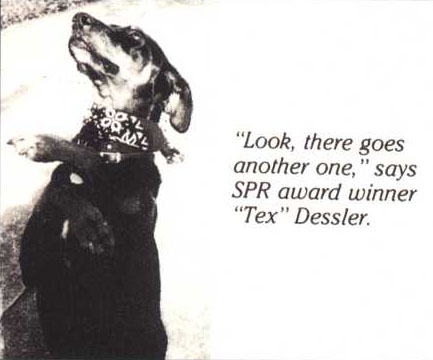
There was one exception to this censure which was found to be distasteful to many of the society’s members. This exception was the dinner award in 1992 to Alexander Dessler’s dog for “keen astronomical observations and loyalty to authority.” The award photograph is shown in Figure 17. But such ridicule is expected in such a hotly contested issue as small comets. I point out to young scientists in all fields that an advance to the frontier of knowledge can often lead to difficult “rites of passage.”
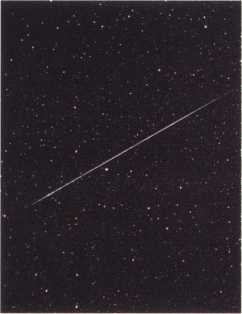
The photograph and caption of Figure 17 does remind one of the mysteries of meteors in our atmosphere. An example of a bright meteor trail is shown in Figure 18. “Conventional wisdom” of the 1970s and 1980s stated that the brightest of the meteors in our atmosphere, or “fireballs,” were due to dense rocks impacting our atmosphere, even though very few were found on the ground at the expected ends of their trails. One such search effort was the Prairie Network located in the midwest U. S. which employed simultaneous sightings by several separated observers and determinations of the point of ground impact by triangulation. Only a sparse handful of meteoritic rocks were found with these searches. A debate over the possibility that the fireballs were due to loosely bound dust rather than the usually accepted iron and stony rocks was never fully resolved.
It is quite possible that the loosely bound “dustballs” are in fact small comets. At the peak times for meteor research 20 to 30 years ago the possibility that these loosely bound objects might be small comets was simply not suggested. After all, the numbers of fireballs with brightnesses similar to or greater than brightest Venus were about 10 million per year, and who would have thought that there were that many small comets impacting Earth’s atmosphere? Crude estimates of the brightness of small comets which are devoid of brightly glowing dust particles are indeed about the brightness of Venus, and their impact rate is about 10 million per year. It is remarkable that the orbits and speeds calculated for many of the “fireballs” are similar to those for the small comets. It would be of great interest if we would return to a new investigation of fireballs with cameras which are able to photograph these objects as seen looking down on the atmosphere from a satellite in low Earth orbit. This would provide a wonderful opportunity to determine the contents of the infalling objects without the obscuration by the atmosphere and the very limited view of a ground observer.
The Moon offered an active battleground of contention. The central argument for interpretation of the Apollo measurements with seismometers placed on its surface by the astronauts is illustrated by [a 1985 “Fluffy” cartoon by W. B. Park in] Figure 19 [Not shown. “Fluffy”, the mascot of several critics of the small comets who insist that the Moon should “ring like a bell”—even if the objects are fluffy small comets—is shown falling through the air like a rock. The caption notes that it doesn’t matter whether Fluffy lands on his feet or not.]. That is, no matter what the impacting object on the Moon was, rock or cat or small comet, the disturbances recorded by the seismometers would be the same. Although amusing, this position is not reasonable when one considers the different results of a hand-thrown rock or snowball, each weighing one pound, for example. It was expected that the Moon would be “ringing like a bell” from the large number of meteors which were causing the fireballs in the Earth’s atmosphere. Surprisingly the Moon was silent, except for a relatively rare meteor event or the thermal groans of the surface.
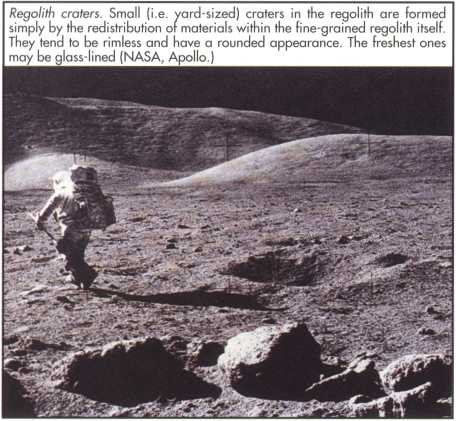
Note the shallow crater to his right.
The ambiguity presented by the numerous fireballs in our atmosphere and the “silence of the Moon” can be resolved with the existence of small comets. The lunar surface is covered by a dust layer with thicknesses typically in the range of feet. This soil layer is called the regolith. The lunar seismometers could easily detect the impact of a stony object because it penetrated through the soil layer to the bedrock and “rang” the Moon’s interior. On the other hand, the impact of a fluffy small comet would produce shallow craters in the lunar soil, not sufficiently deep to penetrate to the bedrock. Because of this shallow penetration and the structural fragility of the small comets, only weak disturbances would be caused by the small comet impacts. Indeed the lunar surface is known to be saturated with shallow craters with diameters of tens of feet which would be expected from numerous impacts by small comets. A photograph of such a shallow crater is shown in Figure 20 at the right-hand side of the Apollo astronaut.
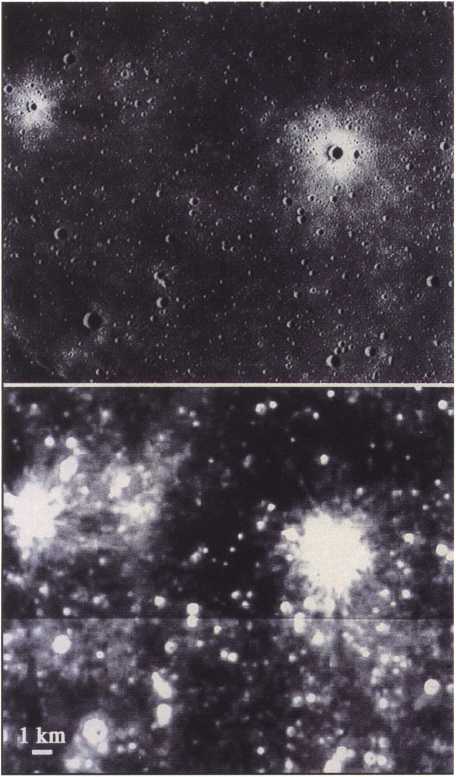
With the Polar spacecraft confirmations of the existence of small comets in three independent ways, there was a new flurry of activity intended to show that their presence was contrary to the lunar cratering record. This evidence was based upon the comparison of Apollo images of the lunar surface in 1972 and images of the same area of the Moon with the Clementine spacecraft in 1994. The comparison of these images separated in time by 22 years in order to search for new impacts on the surface of the Moon due to small comets was a great idea. Unfortunately the work failed as noted by the images shown on the cover of Geophysical Research Letters of December 15, 1997 and reproduced here as Figure 21. At the top of the figure is shown the high resolution Apollo image. A scale bar of 1 kilometer, or 3300 feet, in the bottom image is valid for both images. The dimensions of the shallow craters from the impact of the small comets are expected to be in the range of a few tens of feet at most. The Apollo images are magnificent and do record craters of these dimensions at their limits. The Clementine image of the same area of the Moon is shown in the lower panel of Figure 21. The resolution of this image is so poor that it takes some examination to convince the viewer that the same surface area of the Moon is being examined. To detect craters of the size associated with small comet impacts is hopeless. To hypothesize huge bright spots as the cometary impacts defies reasonable considerations of the actual impacts. It is disappointing that the Clementine images were too blurry for the comparison. Otherwise the results would have been very exciting.
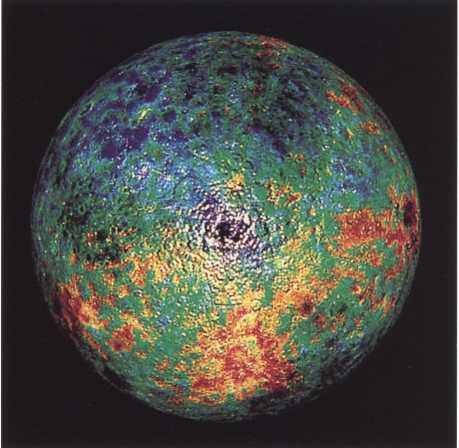
For many years after the original publication of the small comet papers in 1986, a frequent objection to their presence in the vicinity of Earth was based upon reports during the Apollo missions that there was a remarkable absence of water or water snow on the surface of the Moon. The critics claimed that the frequent impacts of the small comets on the lunar surface should have left tell-tale signs of water. Of course, even though the Moon’s gravity is relatively low, in the sense that it cannot prevent the high speed water outflow from the small comet impacts from escaping into space, there should be at least a little water ice or snow which is trapped in the surface crevasses and other shadowed areas of the lunar surface. The lunar surface is cold but any water ice or snow is quickly vaporized by direct sunlight. Last year a remarkable search for lunar water was reported by the Lunar Prospector spacecraft which is currently orbiting the Moon. A sensor on board this spacecraft was capable of remotely sensing the presence of hydrogen-bearing substances. The most likely substance with hydrogen is water. One of the first maps of this water on the lunar surface is shown in Figure 22. The view is the northern polar region of the Moon. The color blue indicates more water and red is lesser quantities. There are many tons of water in the polar region. This fascinating survey continues with the spacecraft orbit being adjusted to achieve lower altitudes and hence to obtain views with better resolution. The Moon is not dry.
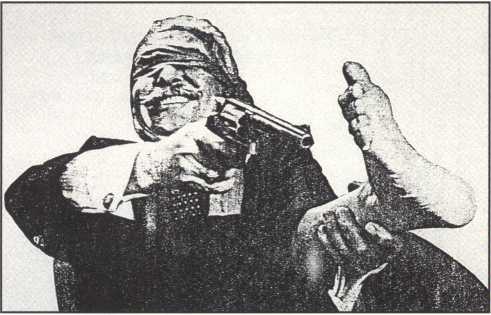
The remaining critics of the small comet theory eagerly awaited the results of the survey of the seasonal variations of the frequency of small comet impacts into our atmosphere with the Polar spacecraft. Years before atmospheric hole rates as determined by the camera on board Dynamics Explorer-1 had been shown to be correlated with radar meteor rates observed with a ground-based station in Canada. The relevant Dynamics Explorer-1 observations were taken during November 1981 through January 1982 and those with the radar during November 1955 through January 1956, some 26 years earlier. The remarkable correlation of the seasonal variations as observed with these entirely different instruments was dismissed as happenstance by the critics. Their responses to these measurements are characterized by the donated photograph in Figure 23. When the seasonal results were reported, and they most certainly were expected to disagree with the previous findings, then we would have shot ourselves in the foot and the small comets would be past history.
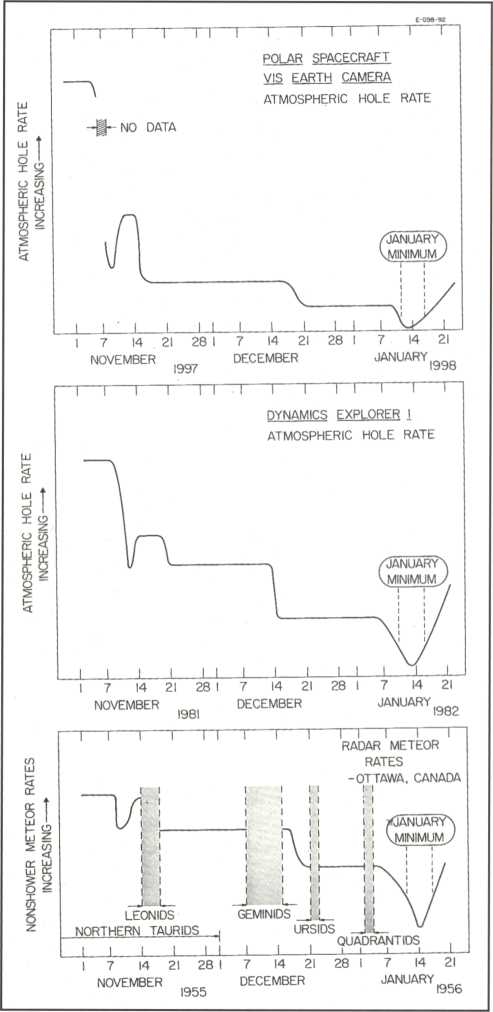
The Polar results were recently reported. Much to the dismay of the critics the seasonal variations agreed with the previous measurements with Dynamics Explorer 1 and the Canadian ground-based radar station. The observations from all three of these instruments are shown in Figure 24. The agreement is truly remarkable and there is no chance it is due to any error. From top to bottom are shown the rates for Polar, Dynamics Explorer, and the Canadian radar. The features of these rates are a maximum intensity during early November, a brief plateau during mid-November, more or less constant rates during late November through mid-December, another plateau of lesser intensities during mid-December through early January, and a well-defined minimum in mid-January with subsequent recovery. There is a further important feature of the radar observations to be seen in Figure 24. That is the large increases of rates associated with the atmospheric impacts of stony and iron meteors during such well-known showers as the Leonids and the Geminids. As expected, because these are very small objects relative to the dimensions of a cometary water cloud, there is no sign of these meteor showers in the Polar or Dynamics Explorer-1 records. The Canadian radar records both the meteor showers due to stony objects and the background events which are due to the infalling small comets.
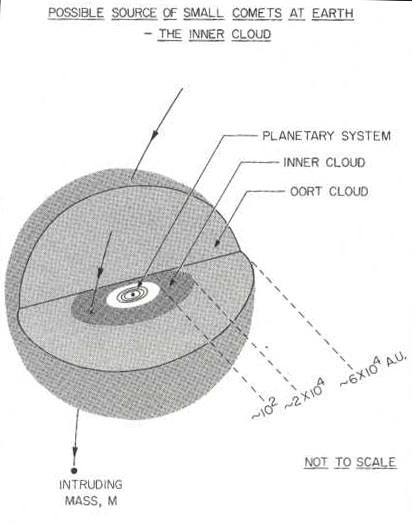
The question arises as to the location of the source of this small comet population which is seen in the vicinity of Earth. We know from the orbits of the small comets, as determined from the speeds of the atmospheric holes and from ground-based telescope observations, that these objects are moving more or less in the ecliptic plane. That is, their motions are in the same general planes as those of the planets. Their speeds at Earth are such that their orbits will carry them to Jupiter’s orbit and beyond. Their origins most likely lie beyond the planets, in a disk of comets which was formed during the birth of our solar system. A diagram of this disk, sometimes called the Oort inner cloud after the famous astronomer Jan Oort, is depicted in Figure 25. This sketch is not to scale for, if it were, the planets would be drawn so close to the Sun as to be not discernible. This disk extends for tens of thousands of Astronomical Units (AU), with 1 AU being the distance of our planet from the Sun. The gravity of passing stars or an as yet undetected dark planet can disturb comets in the disk and scatter them into the inner solar system of planets. Evidence of such a disk of comets, presumably both large and small, comes from the existence of a large spherical cloud of comets, the Oort cloud, which is also indicated in Figure 25. Their original location is thought to be in the inner cometary disk until their orbits were changed by passing large bodies such as stars.
The large numbers of the small comets in the vicinity of Earth provide stimulation of numerous questions on the horizon. Among these are:
What are the organic compounds which are formed in the small comets after they have been bombarded by galactic radiation for billions of years?
Can these small comets safely deposit their organic contents into the atmosphere?
Can these organic compounds be the seeds for life on our planet?
Do some small comets arrive from distant stellar systems after journeys of millions and billions of years?
The final frontier
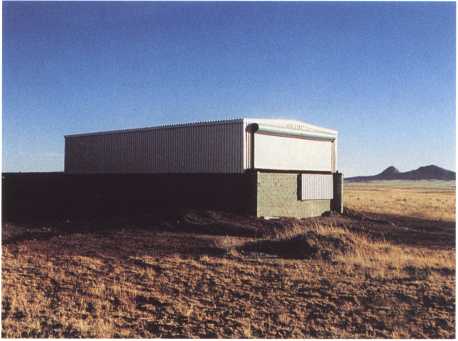
In a desolate place not far from Tombstone, Arizona is a robot telescope from the University of Iowa which was constructed and is operated under the guidance of Robert Mutel, a professor at our university. The building which houses the telescope is shown in Figure 26. This building rolls to the left to allow the telescope to view the sky. This telescope, the Iowa Robotic Telescope (IRO), is seen in the photograph of Figure 27. It is a matter of great coincidence, and perhaps of irony, that this telescope can play a crucial role in the final confirmations of the small comets.
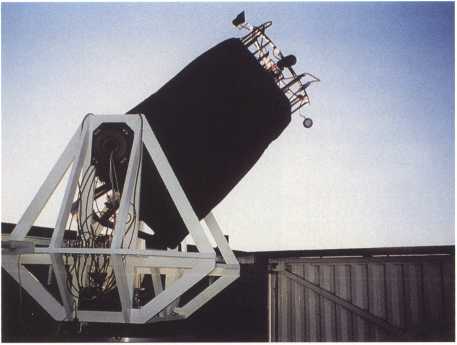
This will not be the first search of the sky for small comets with a ground-based telescope. About ten years ago Clayne Yeates, a scientist at the Jet Propulsion Laboratory in Pasadena, California designed a very clever way of detecting the small comets with the Spacewatch Telescope of the University of Arizona. His method relied upon passage of small comets by the Earth in an organized stream as inferred from the motions of atmospheric holes observed with Dynamics Explorer 1. Clayne, like so many other scientists in the 1980s, did not believe that the small comets existed. His technique to detect these small, dark, fast objects is shown in Figure 28. Telescopes are traditionally pointed so that they are staring at the stars. In order to see the small comets Clayne used the telescope in a “skeet shooting” manner. In other words, the telescope’s pointing was moved in such a way as to keep the small comets in the sights of the telescope for a sufficiently long time that they would be recorded in the images.
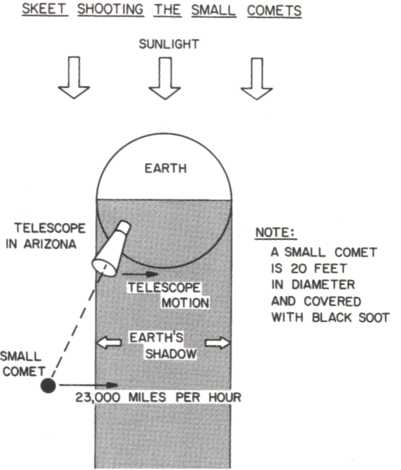
To Clayne’s surprise he in fact did find the small comets in his images and in numbers that were predicted from the observations of atmospheric holes. The small comets were clearly detected in the images. Astronomers insisted that the comets should be detected in two consecutive photographs. Clayne returned to the telescope and gained these pairs of images of each small comet. Astronomers returned with the ridiculous demand that they now needed three images. The small comets were there and the astronomers of the Spacewatch Telescope could only offer the now familiar “camera noise” as a defense. Because of his untimely death, Clayne was not able to continue his brilliant applications of ground-based telescopes in the pursuit of small comets.
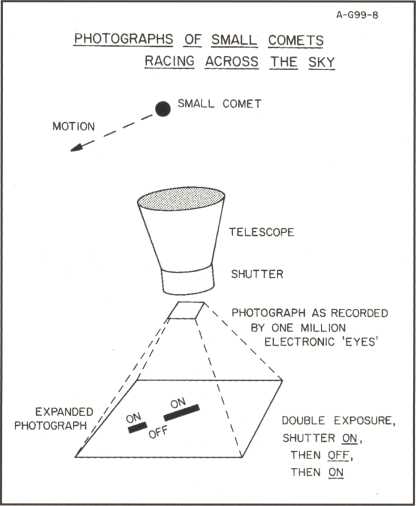
The Iowa telescope is now being used to verify the existence of the small comets. The initial images already are being scrutinized. In order to prevent any further claims of “camera noise” the image of a small comet is taken with two exposures in the same picture. This simple method is shown in Figure 29. The small comet is moving in the telescope’s view. A CCD sensor is placed at the focus of the telescope in order to record the image. These sensors are found in many of the present-day video cameras although the sensor in the Iowa telescope has some special design features. In order to distinguish a real comet signal from “camera noise” two exposures are taken with a mechanical shutter. One of the exposures is twice as long as the other and these exposures are separated by a shutter closed time equal to the short exposure time.
The result for detection of a single small comet is two trails, one about twice as long as the other and separated by a gap with no trail. This is shown in the lower part of Figure 29. The ratios are not quite exact because of the small blurring due to the telescope’s resolution. Also you will note that the trails are shown as dark, rather than bright trails. This inversion of dark and bright is done because the human eye can detect the events more easily in the images.
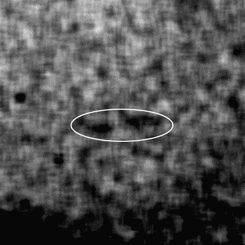
An early candidate image of a small comet which was taken on October 19, 1998 is shown in Figure 30. The mottled appearance of the image is indeed due to camera noise. This picture is presented in order to provide the reader with an accurate accounting of the difficulties in detecting the presence of these small dark comets, and the reasons why these objects were not previously discovered by accident with ground-based telescopes. At the lower border of the photograph is the dark trail of a star. In the center image are the two trails of a single small comet. The analysis of the image proceeds by verifying that the trails conform to the very demanding restraints.
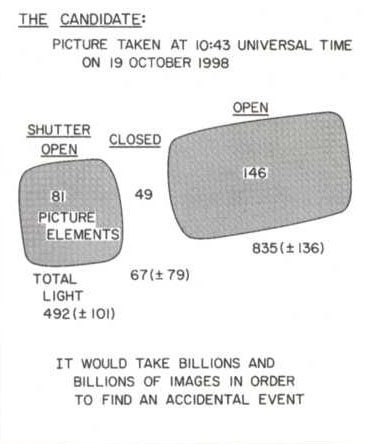
The results of the analysis are shown in Figure 31. It is very exciting to find that the trails recorded by more than 200 individual photoelectric, or “picture elements,” of the CCD conform to the expectations from special shutter operation. It would take billions of such pictures before a “noise event” of this type would occur. We are well on our way for the final confirmation of the existence of small comets. A large number of such detections is necessary to complete the confirmation.
Closing comments
I would like to note that it seems almost incredible that, two scientists, John Sigwarth and myself, from the University of Iowa have participated in the four major milestones in the discovery of small comets. Briefly these milestones are as follows:
1986 | Initial detection of small comet impacts into the atmosphere with the Iowa camera on Dynamics Explorer 1 |
1989 | Direct sightings of small comets with the Spacewatch Telescope of the University of Arizona |
1997 | Confirmation of atmospheric impacts and discovery of disrupting comets with the Iowa cameras on the Polar spacecraft |
1999 | Current search for small comets with the Iowa Robotic Observatory in Arizona |
The scientific debate concerning the existence of small comets has been characterized by intense intellectual and emotional turmoil. I often recall the droll statement attributed to the famous physicist Max Planck who had also experienced considerable criticism from his colleagues.
“Scientists don’t change their minds, they just die.”
References
Key references for the lecture are given in the following list with a short description as to their relevance to the subject of small comets. The papers are listed in approximate chronological order of their publication date. Further relevant papers can be found in the reference lists of these papers.
- The original discovery of the atmospheric holes in the Dynamics Explorer-1 images and their interpretation in terms of small comets: L. A. Frank, J. B. Sigwarth and J. D. Craven, Geophysical Research Letters, 13, 303-310, 1986.
- Detections of the small comets with the ground-based Spacewatch Telescope in Arizona: C. M. Yeates, Planetary and Space Science, 37, 1185-1196, 1989. L. A. Frank, J. B. Sigwarth and C. M. Yeates, Astronomy and Astrophysics, 228, 522-530, 1990.
- A popular trade book describing the small comet controversy and the reactions of the scientific community: L. A. Frank (with Patrick Huyghe), The Big Splash, Birch Lane, New York, 1990, (Reprinted by Avon, New York, 1991.)
- A summary of the criticisms of the existence of atmospheric holes and small comets: A. J. Dessler, Reviews of Geophysics, 29, 355-382, 1991.
- Comprehensive responses to the above criticisms of atmospheric holes and small comets: L. A. Frank and J. B. Sigwarth, Reviews of Geophysics, 31, 1-28, 1993.
- Confirmation of the existence of atmospheric holes with the Polar spacecraft: L. A. Frank and J. B. Sigwarth, Geophysical Research Letters, 24, 2423-2426, 1997.
- Discovery of oxygen trails from small comets at large distances from Earth: L. A. Frank and J. B. Sigwarth, Geophysical Research Letters, 24, 2431-2434, 1997.
- Discovery of water fragments in the water clouds of small comets as they plunge into our upper atmosphere: L. A. Frank and J. B. Sigwarth, Geophysical Research Letters, 24, 2435-2438, 1977.
- Comparison of images of the Moon with Apollo and the Clementine spacecraft in an attempt to find recent impacts of small comets: J. A. Grier and A. S. McEwen, Geophysical Research Letters, 24, 3105-3108, 1997.
- Suggestion that a few of the atmospheric holes may be due to the impact of stony meteors in our atmosphere: M.B.E. Boslough and G.R. Gladstone, Geophysical Research Letters, 24, 3117- 3120, 1997.
- Findings of an unexplained water vapor layer in our upper atmosphere: M.E. Summers and others, Science, 277, 1967-1970, 1997.
- Discovery of water frost on the Moon with the Lunar Prospector spacecraft: W.C. Feldman and others, Science, 281, 1496-1500, 1998.
- Recent advances in studies of the possible influence of extraterrestrial volatiles on Earth’s climate and the origins of the oceans: D.Deming, PALAEO, 146, 33-51, 1999.
- Comprehensive investigation of atmospheric holes with the Polar spacecraft that eliminates the possibility of camera errors and firmly establishes the reality of atmospheric holes and their geophysical effects: L.A. Frank and J.B. Sigwarth, Journal of Geophysical Research, 104, 115-139, 1999.
Acknowledgment
This research was supported in part at The University of Iowa by the National Aeronautics and Space Administration under Contract NAS5-30316 and Grant NAG5-3328.
Web Site
Frequently Asked Questions (FAQs), a variety of images, and contents of related scientific papers can be found at
http://smallcomets.physics.uiowa.edu/
Illustration Acknowledgments
Fig. 1 L. A. Frank, J.B. Sigwarth, and J.D. Craven, Geophysical Research Letters, April 1986;
Fig. 2 L. A. Frank and J.B. Sigwarth, University of Iowa;
Fig. 3 NASA;
Fig. 4 Weekly World News, March 3, 1992;
Fig. 5. J.B. Sigwarth, University of Iowa;
Fig. 6 L. A. Frank and J.B. Sigwarth, Geophysical Research Letters, October 1, 1997;
Fig. 7 L. A. Frank and J.B. Sigwarth, Astronomy Magazine, October 1998;
Figs. 8-9 L. A. Frank and J.B. Sigwarth, University of Iowa;
Fig. 10 L. A. Frank and J.B. Sigwarth, Geophysical Research Letters, October 1, 1997;
Fig. 11 Patrick Huyghe;
Fig. 12 A Teacher’s Guide to the Geology of Hawaii Volcanoes, Hawaii Natural History Association;
Fig. 13 K.Segerstrom, U.S. Geological Survey;
Fig. 14 Source unknown;
Fig. 15 Science News, Vol. 152, No. 8, August 23, 1997;
Fig. 16 Science, Vol. 277, August 22, 1997;
Fig. 17 EOS, Vol. 73, March 7, 1992, American Geophysical Union;
Fig. 18 Akira Fujii;
Fig. 19 Drawing by Park, United Feature Syndicate, Inc. 1985;
Fig. 20 NASA;
Fig. 21 J.A Grier and A.S. McEwen, Geophysical Research Letters, December 15, 1997;
Fig. 22 Web site http://lunar.arc.nasa.gov/science/newresults/ (3D Maps);
Fig. 23 Source unknown;
Fig. 24 L. A. Frank and J.B. Sigwarth, Journal of Geophysical Research, January 1, 1999;
Fig. 25 L. A. Frank and J.B. Sigwarth; University of Iowa;
Figs. 26-27 R.L. Mutel, University of Iowa;
Figs. 28-31 L. A. Frank and J.B. Sigwarth, University of Iowa.