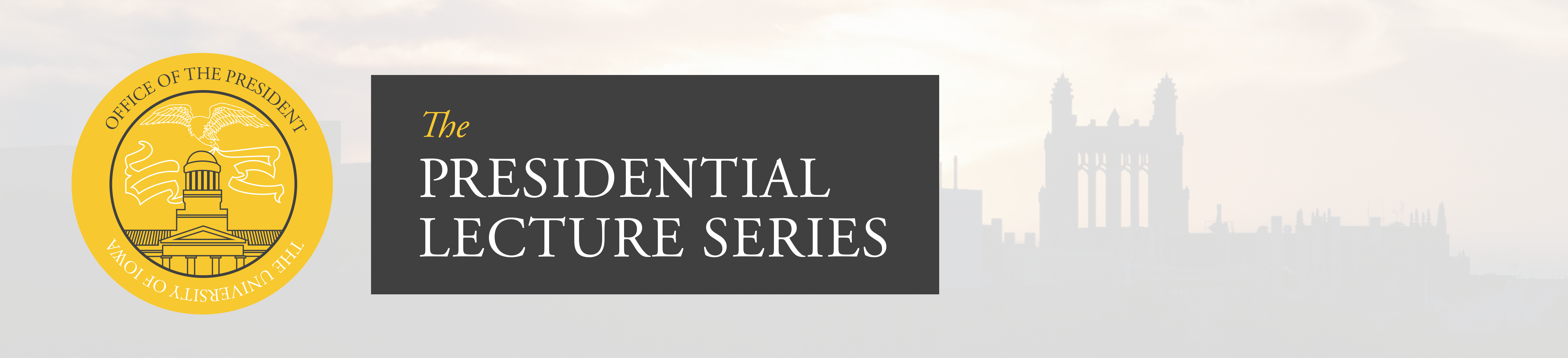
10th Annual Presidential Lecture Series
https://doi.org/10.17077/fwp3-uvp2
O Brave New World!:
Exploring the Mind and Brain in Health and Disease
Department of Psychiatry
The University of Iowa College of Medicine
and
The Mental Health Clinical Research Center
The University of Iowa Hospitals and Clinics
O chestnut tree, great rooted blossomer,
Are you the leaf, the blossom or the bole?
O body swayed to music, O brightening glance,
How can we know the dancer from the dance?
This lecture is dedicated to celebrating the achievements of the human mind and the human brain. One of the remarkable accomplishments of our world and universe, as will be elaborated in this presentation, is the paradoxical capacity of the human mind to study and understand itself.
Philosophical tradition has sometimes, unfortunately, created a dichotomy between the mind and the brain, producing the so called “mind/body problem.” Yet just as, in the lines by Yeats, the dancer and the dance are indistinguishable, so too are the mind and the brain. What we call the mind represents the product of activity within the brain occurring at the cellular, chemical, and molecular levels.
Human beings recognized the link between the mind and the brain as early as the Neolithic period. Skulls survive from Neolithic times that have been trephined, or drilled into; we surmise that the trephining was done as a medical treatment to release evil spirits, as the demons of mental illness or epilepsy have been so often perceived down through the ages. In classical times the eminent physician, Hippocrates, observed:
men ought to know that from the brain, and from the brain only, arise our pleasures, joys, laughter and jests, as well as our sorrows, pains, griefs, and fears. Through it, in particular, we think, see, hear....
The brain forms the essence of what defines us as human beings. To understand its structure and its workings is to understand ourselves. The normal healthy brain is a complex, miraculous, and ingeniously created organ. It permits us to achieve the wonders of music, art, science, architecture, engineering, political organization, and economic structure. Each of us has been endowed with a unique brain with particular capacities that we can either enhance through learning and productive work or waste through intellectual inactivity and unhealthy living habits. We can use our brains to good ends, as when we write poetry or discover radiation belts, or we can use our brains to harm or destroy one another in uniquely innovative ways.
A description of the exciting technological developments that have enhanced our capacity to learn about the workings of the healthy normal brain forms the first part of this presentation. The second part is devoted to an exploration of how the working of the brain is sometimes injured through disease processes, which we refer to mental illnesses. When the normal brain has been injured by disease, we observe some of the most troubling and tragic experiences that human beings can have.
Although the human mind has been intrigued by its brain for centuries, the mind has had the capacity to study the brain scientifically only for a short period of time. Early efforts are represented by the science of anatomy in western culture. The study of anatomy is a relatively recent development, originally initiated by artists such as Michelangelo and Leonardo, who have left us with a variety of anatomical drawings indicating their preoccupation with understanding the structure of the body and the brain. Paintings by Rembrandt illustrate the active study of anatomy during the seventeenth century, including famous portrayals of the dissection both of the hand and of the brain. These early techniques were necessarily limited, however, since they permitted medical scientists to see only on a large-scale level and to investigate only the appearance of the brain after death. The opportunity to study the living, working, thinking brain is a relatively new development in the history of mankind. Most of this effort has occurred during the past one or two decades. It has launched a veritable voyage of exploration, in which investigators have set out to map the human mind and brain in ways that were previously impossible.
In Vivo Neuroimaging
This voyage of exploration has been made possible through the development of a group of techniques that are referred to as “in vivo neuroimaging.” The techniques are summarized in Table 1. In vivo neuroimaging involves methods that permit us to visualize and study the brain during life (i.e., in vivo). Four principal techniques have been developed. The oldest is Computerized Tomography (CT), developed in the 1960s. Its inventors, Hounsfield and Cormack, were awarded a Nobel prize for this achievement in 1979. A related technique, Nuclear Magnetic Resonance Imaging or more simply Magnetic Resonance (MR), came to the forefront in the 1980s. Purcell and Bloch, who discovered the phenomenon of nuclear magnetic resonance, were also awarded a Nobel prize. CT and MR both permit us to see the anatomical structure of the brain in fine detail. Because its spatial resolution is much greater, MR has now largely supplanted CT as a scientific investigative tool, although CT remains clinically important.
TABLE 1
In Vivo Neuroimaging Techniques
Structural Techniques (Anatomy)
- Computerized Tomography (CT)
- Magnetic Resonance (MR)
Functional Techniques (Chemistry, Metabolism)
- Single Photon Emission Computed Tomography (SPECT)
- Positron Emission Tomography (PET)
The second major group of neuroimaging techniques encompasses the “functional techniques.” These include Single Photon Emission Computed Tomography (SPECT) and Positron Emission Tomography (PET). These methods permit us to observe the brain while it is actually functioning-thinking, remembering, seeing, hearing, imagining, or experiencing pleasure or displeasure. The two groups of techniques complement one another very nicely. Structural techniques permit us to see the brain in fine detail and to perceive how various parts are related to one another spatially. The functional techniques permit us to examine how the brain responds to stresses or challenges by changing its metabolism and blood flow. When the two groups of techniques are combined together, we can visualize how the brain works as we never could before. We can map virtually unexplored territories in human brain development and human cognition.
It is a great privilege to be able to do scientific research in an era when these technologies have become available. I went into medicine and psychiatry in the hope that I would be able to understand the mind and to develop ways to understand and to help people suffering from serious mental illnesses. In its early stages, the effort was often arduous and discouraging. As CT, MR, SPECT, and PET have become available, I and other scientists have experienced new hopes that we will truly be able to understand the brain and its abnormalities. This endeavor is perhaps one of the most exciting voyages of exploration that a scientist could undertake. Like the explorers of space, we are moving through regions where no man or woman has gone before. Each day begins with curiosity and excitement, and with the recognition that we will be surprised and delighted by our inability to predict what we can find, coupled with our capacity to find something. It is also a privilege to work here at Iowa, where the excellent medical and hospital infrastructure and interdepartmental cooperation have permitted me to have access to these neuroimaging techniques. Iowa has state-of-the-art equipment in all these modalities and offers one of the best opportunities in the world to apply the study of neuroimaging to the understanding of the mind and brain in health and disease.
Development of Imaging Analysis Techniques
and the Study of the Normal Brain
Medical imaging techniques such as MR were designed primarily for medical visualization: simply to recognize lesions (abnormalities) in various parts of the body. Turning clinical probes into scientific probes requires innovative adaptations. One of the major efforts of our research group has been to develop methods that will permit us to use medical imaging in order to measure, to quantify, and to answer fundamental questions about brain structure and function. One important component of our effort, therefore, has been the creation of computer software to achieve these ends. This is represented by a locally-developed family of computer programs which we refer to BRAINS (an acronym for Brain Research: Analysis of Images, Networks, and Systems). The various utilities in this program and the image analysis problems that they solve are summarized in Table 2. This set of programs, developed by the talented computer scientists on our research team, permits us to apply neuroimaging techniques to answering a variety of important questions about the nature of the normal human brain. For example, how does the brain change during the aging process, or does it change at all? Are there relationships between brain structure and mental abilities, such as general intelligence? What are the relative contributions of genetic factors and environmental influences to the development of the brain and of mental capacities?
TABLE 2
A Comprehensive Neuroimaging Software Package: BRAINS
(Brain Research: Analysis of Images, Networks and Systems)
Program | Purpose |
---|---|
BRAINBLAST | A voxel processing program that permits three-dimensional visualization, resampling, tracing on multiple views, and identification of sulci and gyri. |
BRAINVOL | Volume measurement |
BRAINCLASS | Automated tissue classification (gray, white, and CSF) |
BRAINPLOT | Quantification of surface complexity |
BRAINMAP | Mapping of sulcal/gyral patterns and quantitative measures of surface area |
BRAINFIT | Registration and resampling of MR and PET images |
BRAINFLOW | Flow measurements, image math |
BRAINMORPH | Transformation of images to standard atlas format |
BRAINNET | Statistical mapping of areas of activation |
One of the earliest programs that we created is referred to as BRAINCLASS. We designed this program because we wanted to know about the relative quantities of different kinds of brain tissue. The brain itself contains two visually discernible kinds of tissue, called gray matter and white matter. The gray matter portion of the brain is composed of the cell bodies (or neuronal “executive centers” that contain the cell’s nucleus), while the white matter contains the axons (or “wires” that connect various cell bodies to one another). Nerve cell bodies are surrounded by dendrites (”tree branches”), which permit neighboring cells to talk to one another and which comprise part of the gray matter, while the axons permit cells to talk to one another over longer distances. This brain tissue is surrounded by cerebrospinal fluid (CSF), which covers its surface and fills internal cavities in the brain (the ventricles). CSF cushions the brain and contains nutrients and metabolic breakdown products. The gray matter portion of the brain includes the cortex (”bark”), or the gray matter on the brain surface that has a bark-like appearance, as well some specific regions inside the brain that have been given rather poetic Latin or Greek names by early anatomists (e.g., hippocampus, or sea horse; amygdala, or almond; thalamus, or marriage bed). (See Table 3.) Because of the complex interconnections between gray matter and white matter, it is impossible to dissect apart these various tissue components in the anatomy laboratory. Prior to the existence of neuroimaging we were unable to answer accurately such simple questions as, what proportion of the brain is composed of gray matter, white matter, or cerebrospinal fluid?
TABLE 3
Anatomy for Literati
Brain Region | Greek/Latin Name | Function |
---|---|---|
Hippocampus | Sea horse | Memory |
Amygdala | Almond | Memory |
Caudate | Tail | Emotion, Motor Modulation |
Putamen | Stone | Motor Modulation |
Thalamus | Marriage bed | Central switchboard connecting many subregions |
Limbic lobe | Border region | Emotion |
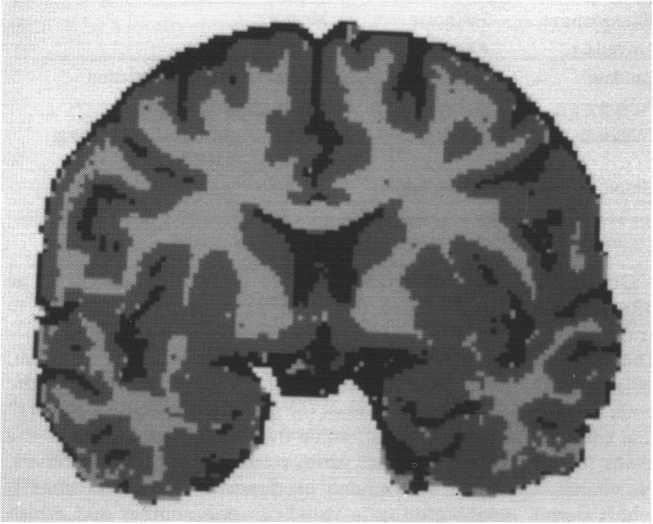
BRAINCLASS is a computer program, which we created in order to classify or “segment” the various types of brain tissue, using informationcollected through magnetic resonance imaging. The data in a magnetic resonance image consist of numbers reflecting tissue properties in small chunks of tissue, called volume elements or “voxels.” Each of these voxels is assigned a gray scale value and is shown as a picture element, or “pixel,” in the MR image, which can be thought of as a dot matrix picture derived from the pixels and voxels. BRAINCLASS uses a statistical technique, discriminant function analysis, in combination with information about small samples of tissue traced on the scan by a technician, and subdivides the continuous gray scale value into three classes or categories: gray matter, white matter, and CSF. An example of a segmented image is shown in Figure 1. While we recognize that the classification is not perfect, we believe that it is sufficiently accurate to answer a variety of important questions about the normal brain.
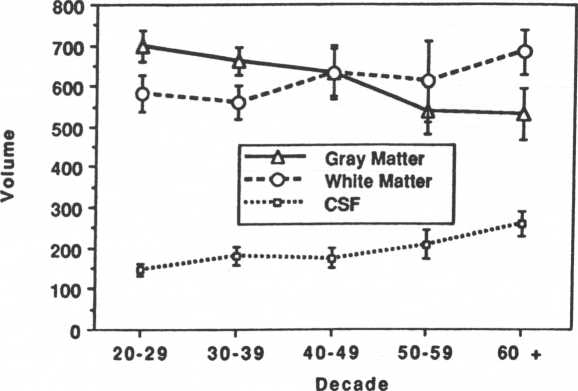
Several examples of information obtained from BRAINCLASS are shown in Table 4 and Figure 2. For example, what happens to our brains as we age? Unfortunately, as Figure 2 indicates, as we grow older, the gray matter in our brain appears to gradually decrease, probably reflecting either cell loss or loss of complexity in the dendritic connections. As the brain shrinks as a consequence of the gray matter loss, the CSF steadily increases. There are many additional interesting questions that we can answer in the future about brain cell loss or brain cell injury using this method. What are the effects of alcohol consumption on the brain? What are the effects of starvation? Are there any ways that we can arrest this process of gray matter loss through exercising our brains intellectually or making our living habits healthier?
TABLE 4
Correlation between IQ Scores and Gray, White,
and CSF Volumes in Normal Controls (N = 70)
Pearson Partial Correlation Coefficients (r) (with height partialled) | |||
---|---|---|---|
Gray Matter | White Matter | CSF | |
Verbal IQ | 0.31** | 0.12 | 0.03 |
Performance IQ | 0.32** | 0.14 | -0.09 |
Full Scale IQ | 0.35** | 0.14 | -0.02 |
** p < 0.01
A related question concerns the relationship between intellectual abilities and gray matter volume. As youngsters, we have jokingly said that particularly bright students have “lots of gray matter.” As Table 4 indicates, this statement may be no laughing matter. There are modest correlations between intelligence, as measured by a standard IQ test (the Weschler Adult Intelligence Scale or WAIS), and gray matter volume. No significant correlations are noted between IQ and volume of white matter or CSF.
Recent improvements in MR technology now permit us to collect information about the brain in very thin slices-in the range of 1.5 mm. It is very difficult to slice the brain in such thin sections in an anatomy laboratory. Using another utility that we have developed, a voxel processing program (colloquially referred to in computer science as “voxel blasting”) called BRAINBLAST, we can generate three-dimensional images of the brain that appear to mirror closely the actual brain. Figure 3 shows an image of a post mortem brain that we have scanned using MR, with the reconstructed image generated by BRAINBLAST adjacent to it. The recreated image and the actual brain are nearly identical. In addition to being able to see clearly the external surface of the brain, with its complex furrowed pattern (called sulci and gyri-or valleys and hills), we can also reslice or “resample” and revisualize the brain in any plane. This enormously expands our capacity to collect information concerning the volume of the brain and its subregions, as well as to make comparisons between individuals. We have only had the capacity to do such reconstructions for the past year or two, and we are just beginning to mine the information that can be generated from these accurate three dimensional reconstructions and resampled images.
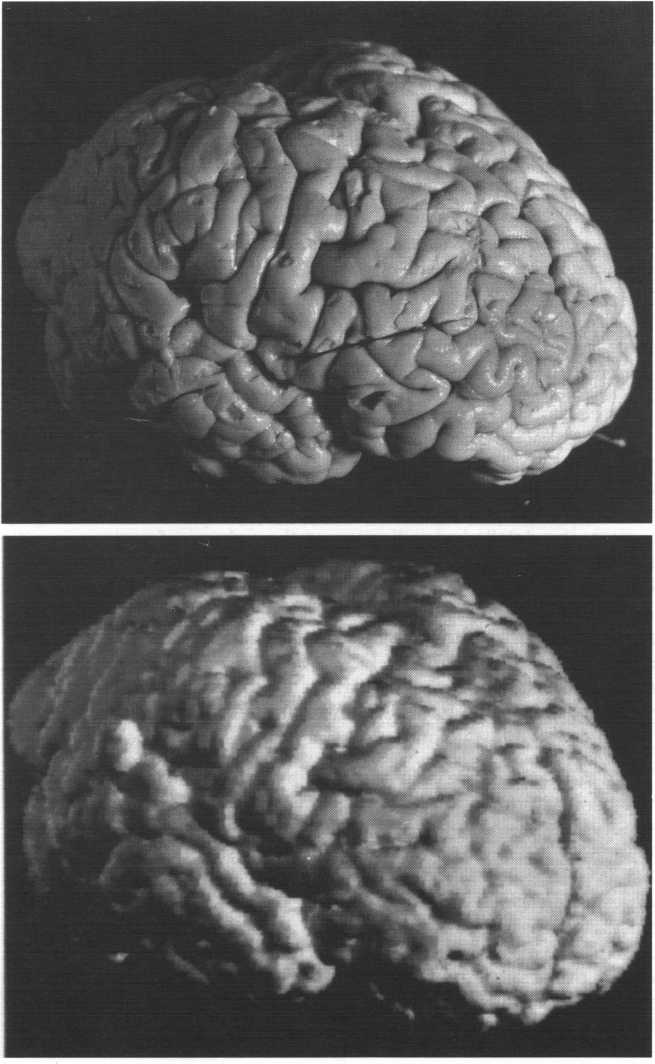
One example of the application of three-dimensional volume measurement is shown in Table 5. This illustrates an additional perspective on the question of the relationship between intelligence and brain structure in normal individuals. While we have already seen that there is a correlation between IQ and the volume of gray matter, the detailed visualization of the volume of various specific structures deep inside the brain permits us to determine which parts of the brain may be particularly important for intellectual functioning. As Table 5 indicates, there is a high correlation between overall brain size and IQ. In addition, there are significant correlations between some structures (temporal lobes, hippocampus), but not others (caudate, putamen, lateral ventricles). The temporal lobes and hippocampus make an important contribution to language and memory, which are significant components of general intelligence.
TABLE 5
Correlations between IQ and Volumes of Specific Brain Structures
in Normal Controls (N = 67)
Pearson Partial Correlation Coefficients ® (with height partialled) | |||
---|---|---|---|
Structure | VIQ | PIQ | FSIQtd> |
Cerebrum | |||
Left | 0.35** | 0.26* | 0.36** |
Right | 0.37** | 0.28* | 0.38** |
Temporal Lobes | |||
Left | 0.34** | 0.2 | 0.33** |
Right | 0.50** | 0.30* | 0.46** |
Cerebellum | 0.38** | 0.41** | 0.44** |
Hippocampus | |||
Left | 0.41** | 0.33** | 0.42** |
Right | 0.32** | 0.23 | 0.32** |
Caudate | |||
Left | 0.03 | -0.01 | 0.02 |
Right | 0.13 | 0.06 | 0.11 |
Lateral Ventricles | |||
Left | -0.01 | 0.07 | 0.02 |
* p < 0.05
** p < 0.01
We can also explore issues such as the differential impact of genetic versus environmental factors on brain structure and function. (As used in discussions of genetic influences, the term “environmental” is broadly defined and includes such factors as the mother’s eating habits while the fetus is developing, the impact of educational experience, or use of alcohol or drugs.) We are currently exploring this issue by examining the brains of a series of identical twins. Identical twins have essentially the same genes, or DNA. To the extent that brain structure and function are completely genetically programmed, their brains should be identical. To the extent that their brains differ, environmental factors must play a role in brain development. Environmental factors include those that are more physical (e.g., illnesses, head injuries, diet), as well as those that are more psychological (e.g., education, stress). Identical twins usually have faces and bodies that are extremely similar, so much so that they are difficult to tell apart. They often help us differentiate themselves from one another through differences in clothing and hair style. What predictions would we make about the similarity or difference between their brains? Will they be more like their faces-highly genetically programmed, but with some environmental input-or will they be more like their hair or clothing-more influenced by the environment and very much under personal control?
The answers are still coming in on this issue, but preliminary work suggests that brains of identical twins differ somewhat in structure and more substantially in function. Comparing three-dimensional reconstructions from MR scanning, obtained from a pair of identical twins from the Iowa City area who have generously served as normal volunteers for our research, we find some differences in the sulcal and gyral patterns of their brains. (See Figure 4.) The fact that they differ at all is significant, because sulcal and gyral patterns are reflective of brain growth early in life. The human brain is essentially smooth until approximately the sixth month of fetal life. At that point, massive expansion in dendritic connections begins to occur, and the brain begins an explosive extension within its confined cavity, the skull. The only way it can continue to grow within this cavity is to crinkle and fold. Different patterns and degrees of crinkling and folding may provide clues as to the kinds of growth that occur at a particular period in time and the impact of various environmental factors on brain growth. For example, if one twin in an identical pair has different patterns of furrowing in a particular region, we can potentially use that information to track back and try to figure out why that region differs, based on what we know about different exposure to various environmental factors. I describe this research, for which the answers are not in yet because the methods for visualizing the brain surface are so new, since it illustrates well the sense of adventure and excitement that arises from exploration of the brain.
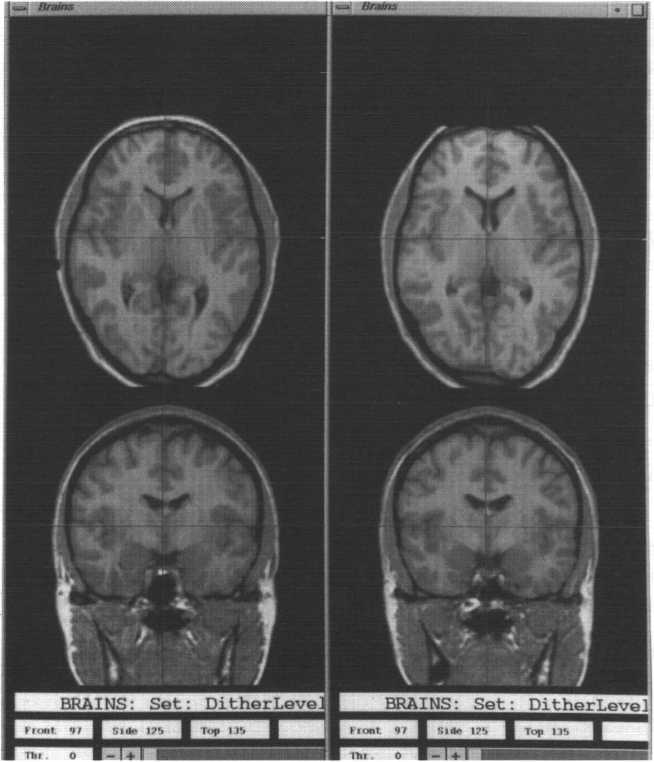
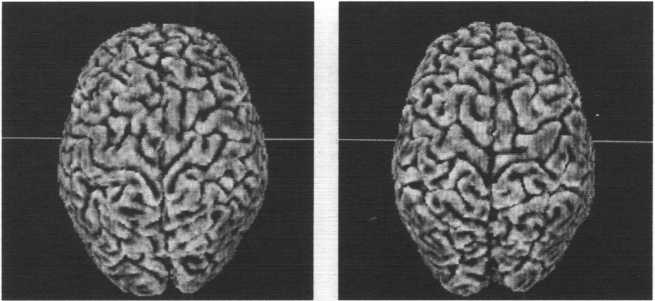
When we compare the brains of identical twins using a functional imaging technique, Positron Emission Tomography, we see differences that are even more striking, as we might expect. Let’s look, for example, at the nature of learning, the fundamental process to which institutions such as the University of Iowa are dedicated. When one twin in an identical pair learns to recognize a set of faces and later is asked to remember them while we measure brain blood flow using Positron Emission Tomography, she uses somewhat different brain regions from her co-twin, reflecting the fact that each of us tends to apply his or her own individual methods for performing mental activities such as remembering. This is illustrated in our two Iowa identical twins, Donna and Deanna, in Figure 5. Twins may begin with the same DNA, but they are shaped by the world around them as well, and they have the wonderful human capacity to shape and mold their lives (and secondarily their brains) through the use of their own personal free choice. The fact that we as human beings have a capacity for choice that affects the development of our minds and brains conveys an important message of hope, as well as an important warning.
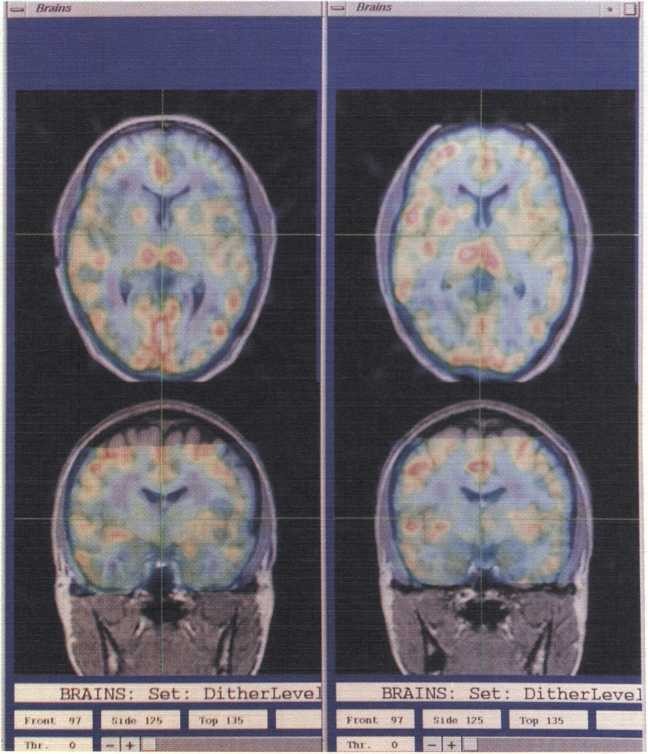
The Mind and Brain in Mental Illness
Having examined some things that we have learned about the healthy normal brain, let us now turn to what we have learned about the effects of disease on the brain. For this part of the presentation, I would like to focus on one particular disease, the very serious mental illness known as schizophrenia.
Schizophrenia can be one of the most tragic of the various mental illnesses, and perhaps one of the most tragic among the broad range of illnesses that affect human beings. The average layperson is often confused about what schizophrenia is. The original name for the disorder was “dementia praecox,” which means a serious illness that affects mental function and begins early in life. It was given this name when it was identified at the turn of the century, in order to differentiate it from a related illness, dementia in the elderly, which later came to be known as Alzheimer’s disease. Both these diseases were identified and differentiated from one another at about the same time in one of the greatest departments of psychiatry of all time, that of Emil Kraepelin in Munich. To the average person Kraepelin’s name is unfamiliar, while the name of his protégé, Alzheimer, survives, because it is used to designate another important mental illness. Schizophrenia would probably best be named Kraepelin’s disease, but instead we call it schizophrenia, which was chosen a few years later by the Swiss psychiatrist, Bleuler, in order to reflect the fact that the illness produces a fragmenting (”schizo”) of the various components of the mind (”phrenia”).
Schizophrenia is a tragic illness, because it typically begins early in life, produces serious incapacity, and persists chronically. A small percentage of patients with schizophrenia do very well and recover almost completely after an initial episode, but the majority have severe social and emotional difficulties that are present for the remainder of their lives. Common symptoms of schizophrenia include hearing voices when no one is around (hallucinations), feeling persecuted (delusions), and exhibiting disorganized speech, disorganized behavior, loss of interest, loss of energy and drive, loss of fluency of thought and speech, and impaired attention. These symptoms are sometimes divided into two broad groups, the positive symptoms, which represent an exaggeration or distortion of normal functions, and the negative symptoms, which represent a loss or diminution of normal functions. The various positive and negative symptoms, and the corresponding functions that are exaggerated or diminished, are summarized in Table 6. One of the major efforts in modern research in schizophrenia is to attempt to link these various symptoms of schizophrenia to the underlying mechanisms that produce them in the brain.
TABLE 6
Positive Symptoms
Symptom | Function Distorted |
---|---|
Hallucinations | Perception |
Delusions | Inferential Thinking |
Disorganized Speech | Language |
Disorganized Behavior | Behavioral Monitoring |
Negative Symptoms
Symptom | Function Lost |
---|---|
Alogia | Fluency of Thought and Speech |
Affective Blunting | Fluency of Emotional Expression |
Anhedonia | Hedonic Capacity |
Avolition | Volition, Drive |
The fact that schizophrenia is a serious and common mental illness, affecting approximately one percent of the population, has been recognized by medical experts for some time. That it is due to disruptions in brain function has not been so widely appreciated, until neuroimaging research resoundingly demonstrated this to be the case during the past ten to fifteen years. CT provided the first major contribution to this recognition. The first study demonstrating that abnormalities in brain structure were present in a group of schizophrenic patients appeared in 1976 in the medical journal Lancet.
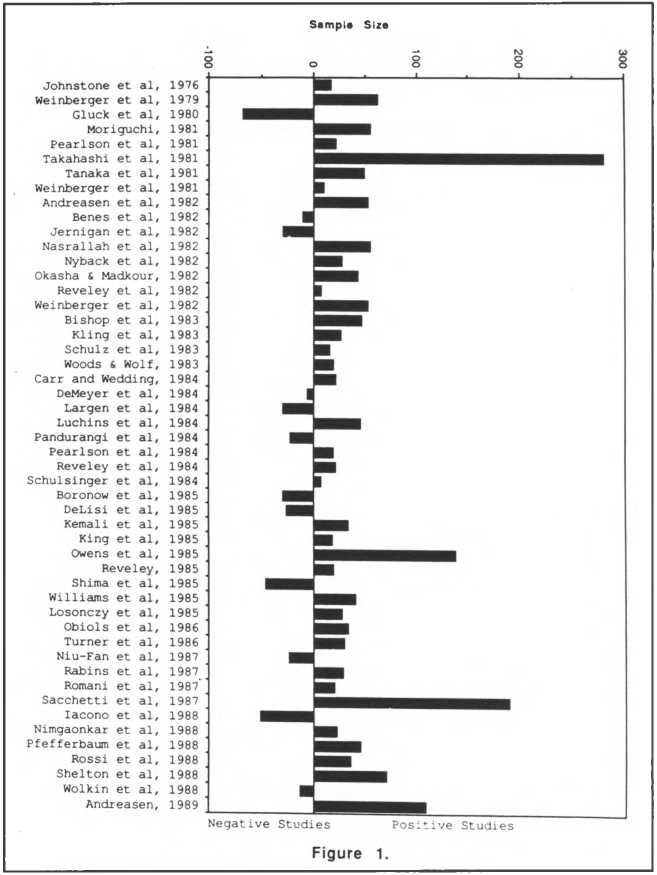
At that time, we had had a CT scanner here at Iowa for several years, and I had tried previously to interest a number of my colleagues in using CT to study the brain in schizophrenia. I had been generally met with disinterest and a comment such as “if there were anything structurally wrong with the brain in schizophrenia, it would have been widely recognized long ago.” The 1976 Lancet study was also greeted with doubt. Fortunately, I persisted, won over my colleagues locally, and was still able to become a pioneer in the application of neuroimaging to the study of brain disease. As Figure 6 illustrates, over the years a large CT database has accumulated which vindicates my early hypothesis about brain abnormalities and the original Lancet study. Approximately 75 percent of the studies published have shown that there are significant group differences in the size of the ventricular system in patients with schizophrenia, as compared with normal controls.
Since enlargement of the ventricles probably reflects some type of broadly-defined brain injury (either failure of the brain to grow and develop correctly or loss of nerve cells or dendrites), these findings suggest that patients with schizophrenia have had some type of insult to their brains that is related to their illness. It should be stressed, however, that these results are neither specific nor diagnostic. Ventricular enlargement is seen in other illnesses, such as Alzheimer’s disease, and it is not present in every person who has schizophrenia. The major importance of this finding is that it has directed medical science to the study of the brain in schizophrenia by indicating conclusively that some kinds of brain abnormalities are found in some patients who suffer from schizophrenia.
Once that discovery was firmly established, the next step was to attempt to find out how and why brain abnormalities occur in schizophrenia. For this effort, magnetic resonance and the functional imaging techniques have provided the most important contributions, since they offer better anatomic resolution and a better opportunity to study physiological and neurochemical dysfunctions.
MR permits us to see small structures in detail. For example, we have suspected for some time from research using postmortem brains that some patients with schizophrenia have abnormalities in the hippocampus. CT does not have sufficient resolution to permit us to visualize the hippocampus, but MR does. As of 1993 there are approximately 10 studies that have demonstrated abnormalities in the hippocampus, the temporal lobes, or sub regions of the temporal lobes such as the superior temporal gyrus, in patients suffering from schizophrenia. Work by our group and a group at Johns Hopkins University has shown that patients who suffer from auditory hallucinations are more likely to have a decreased size of the superior temporal gyrus, an important brain area for auditory perception in normal individuals. Work of this type illustrates the power of neuroimaging to link the specific symptoms of schizophrenia to their specific underlying brain abnormalities.
Another brain region that has been of great interest in the study of schizophrenia has been the prefrontal or frontal cortex. The frontal cortex occupies approximately one third of the cortical area in the normal human brain. This region, on the other hand, is relatively small in various so-called “lower” animals, such as mice, cats, dogs, or chimpanzees. Most cognitive neuroscientists believe that the expansive growth of the prefrontal cortex in human beings provides the foundation for the various human capacities that differentiate us from these lower animals. We are the only creatures on earth who write novels and poetry, play organs and violins, build bridges and highways, design sailing vessels and Explorer spacecraft, or for that matter study the mind and brain with neuroimaging.
The functions of this important area in the human brain are still being delineated. Some of its well-recognized functions are summarized in Table 7. They include fluency of thought and speech, formation of emotional attachments, social judgment, volition and drive, planning and identifying goals, temporal sequencing of behavior, and focusing of attention. Interestingly, these frontal functions bear a striking resemblance to the functions that are lost or diminished in patients with schizophrenia who suffer from negative symptoms. Consequently, the exploration of the relationship between the frontal cortex, the presence of schizophrenia, and the presence of negative symptoms has been a major focus of psychiatric research during the past several years.
TABLE 7
Frontal Functions
Fluency of thought and speech |
Affective attachments |
Social judgment |
Volition and drive |
Planning and identifying goals |
Temporal sequencing of behavior |
Attention |
We have recently completed a very interesting study of the prefrontal cortex using Single Photon Emission Computed Tomography (SPECT). Although this is a relatively crude technique compared to Positron Emission Tomography (PET), we began this project when PET was not available to us, and employed an innovative design that permitted us to stretch this relatively inexpensive and accessible neuroimaging technique to its scientific limits. We were interested in determining whether patients with schizophrenia had decreased frontal function, whether this could be elicited by stressing the frontal cortex by asking subjects to perform a particular mental task, whether decreased frontal function (”hypofrontality”) was present early in the illness or whether it was secondary to having been ill for a long time (”chronicity”), whether it was possibly produced by medications used to treat schizophrenia, and whether it was related to negative symptoms. Consequently, we studied three groups of people: healthy normal volunteers, patients with schizophrenia who were admitted to the hospital for their first episode of illness and had never been treated with any medication, and patients who had been chronically ill with schizophrenia and who were evaluated two times, once while on medications and once after they had been withdrawn from their medications for a three week period of time. In this study we used a cognitive stimulation task, the Tower of London, which requires that the individual be able to make a sequential plan to move a series of colored discs in order to reach a final goal pattern. Their cerebral blood flow while performing this task was compared to that observed during a baseline condition, which involved watching an undulating color shape on a video monitor. We predicted that the undulating color shape would produce activation of the back of the brain (occipital cortex), where visual perception occurs. We predicted that the Tower of London would activate the occipital cortex as well (since it involves visual perception), but would also stimulate prefrontal regions involved in sequential planning.
The results of this study have been important and useful in telling us more about the nature of schizophrenia. Essentially, we observed that impaired frontal function was already present in people who had been hospitalized for schizophrenia in its beginning stage (the first-episode patients). While performing the Tower of London, they were unable to activate their frontal cortex as much as healthy normal volunteers. In addition, the patients with schizophrenia who had been chronically ill were also unable to activate their frontal cortex while off medication, and their degree of hypofrontality was similar to the never-treated first episode patients. When they were treated with psychoactive medication, however, they did show some improvement in frontal blood flow while performing the Tower of London. From this study we have been able to reach a number of important conclusions: that the frontal lobes function poorly in untreated schizophrenia, that this malfunction is not caused by treatment (since it occurs in never-treated first episode patients), that it is not due to chronic illness, that it is associated with prominent negative symptoms, and that it may be partially reversible with medication. Studies of this type permit us to continue to identify brain regions involved in schizophrenia, and as well they suggest that the medications used to treat the illness may help reverse underlying anatomic, neurochemical, or metabolic deficits. Ultimately, we hope that studies of this type will assist in finding more effective medications that can reverse deficits more completely.
A final neuroimaging tool that we are just beginning to apply to the study of schizophrenia is Positron Emission Tomography. This is a very powerful technique, in that it permits us to take a series of sequential “snapshots” of the brain while it is performing mental activities during relatively short time windows-from 20 to 40 seconds. We can do as many as 8 to 10 back-to-back studies of the same person within a two to three hour period. Using this technique, each individual can serve as his or her own control, thereby reducing the individual variation that occurs when people must be compared to one another in groups. Because one can examine a large number of conditions in a single imaging session, we now have the opportunity to dissect apart the various components that are involved in mental activities. For example, we can compare the brain regions involved in short term versus long term memory, or memory for faces versus word lists. We can determine whether different brain regions are involved when the brain hears environmental sounds such as horns honking versus words that are combined together in sentences. We can explore whether perceiving language involves different mental activity from generating language. The list of things that we can study is endless and exciting.
Our efforts with Positron Emission Tomography are currently moving in two directions. We have begun by exploring a variety of mental functions in healthy normal volunteers. We have selected some of the most fundamental of human mental activities: language, memory, attention, and the experience of pleasure. We have also selected these because all these functions are disrupted in serious mental illnesses such as schizophrenia. Therefore, the data accumulated concerning normal brain function can be used to study disruptions in schizophrenia. This work is currently underway, using the excellent PET center that has been available to us for the past year.
I would like to illustrate some of this work by choosing an example from one study, which is focusing on the nature of pleasure. We have chosen to study pleasure because the loss of the capacity to experience pleasure, or anhedonia, is one of the most important negative symptoms of schizophrenia. Young people who develop this illness often complain that they seem to have lost the capacity to feel. Love for their family members, the ability to enjoy athletic activities, or joy in relating to friends all seem to have been stolen from them, and they experience a tragic sense of loss. This symptom, anhedonia, is also very painful for parents to witness, since they too share their child’s sense of loss.
TABLE 8
What is Pleasure?
Appetitive satisfaction—fulfillment of basic drives and appetite – e.g., sex, food |
Interpersonal satisfaction—drive to reduce existential loneliness through love and intimacy |
Success—drive to establish a sense of identity, autonomy, and the capacity to achieve excellence |
Playfulness—drive to experience and absence of constraints |
Creativity and Exploration—drive to discover, invent, satisfy curiosity, answer questions |
Power—drive to establish superiority and dominance |
Tranquility—drive to experience peace and lack of striving |
Altruism—drive to satisfy an ideal image or value through helping others |
Pleasure is in fact a complex function. It ranges from the lowest of elements, such as the appetitive drives (eating, sleeping, sexual experience), through higher human functions such as creativity or altruism. Wittgenstein has reminded us that “just because there is one word for a thing does not mean there is only one thing.” That is certainly the case with pleasure. Table 8 lists some of the many diverse types of pleasure that human beings experience.
In our PET studies, we have focused on three elementary forms of pleasure for our initial work, since in science it is best to begin things at a relatively simple level before progressing to more complex questions. In this preliminary work, we have attempted to determine whether three different types of pleasure have a common center in the brain, or whether they are diversely represented. In our pleasure paradigm, the three elements that we have studied so far include smell, taste, and music; we have given the subject both a pleasant and an unpleasant stimulus, so that we can compare the differential effects of pleasure and displeasure on the brain.
Traditional teaching has suggested that there is a “pleasure center” in the brain. Our PET studies to date suggest that the story is likely to be much more complicated. Although the “pleasure center” has been postulated to be buried deep inside the brain in midline structures, especially the limbic system, our work suggests that many aspects of pleasure (even elementary pleasure) may instead be represented in the cortex, or surface of the brain, where many higher intellectual activities are performed. We have also observed that unpleasant stimuli seem to produce an even higher degree of brain activity than pleasurable stimuli-a somewhat poignant commentary on our fate as human beings. As this basic groundwork concerning understanding of the normal brain progresses further, we will begin to apply it to the study of anhedonia in schizophrenia, in order to determine how and why this terrible loss of the capacity to experience pleasure has occurred in patients with this illness.
Past, Present, and Future
Human beings moved out of caves and began to cultivate the soil thousands and thousands of years ago. They began to record their history in written language four to five thousand years ago. In this context, the scientific study of the human mind and brain is extremely youthful. It has only been underway for a mere 100 to 150 years. The neuroimaging techniques I have described have been available for only one or two decades. The progress that has been achieved in that short period of time is astonishing and is also a cause for great hope. As this work continues, it seems likely that we will increasingly be able to understand how the mind and brain work in health, illuminating the miracles of intelligence, learning, and creativity. Armed with the knowledge that we have acquired, we hope and believe that we will ultimately be able to find new ways to heal serious mental illnesses such as schizophrenia.
O,
wonder!
How many goodly creatures are there here!
How beauteous mankind is! O brave new world,
That has such people in’ t!
Acknowledgements
The research effort described here represents contributions from a large number of colleagues from a variety of disciplines. I would like to express my appreciation in particular to the College of Medicine and especially to the Department of Radiology, to the University of Iowa Hospitals and Clinics (UIHC), and to my colleagues in the Mental Health Clinical Research Center and the Department of Psychiatry. The College of Medicine has been my intellectual home for more than two decades, providing first an excellent education and subsequently sustained intellectual support. John Colloton of UIHC has been particularly generous in providing excellent facilities for the Mental Health Clinical Research Center, as well as the technical infrastructure of the hospital as a whole and of its state of the art imaging equipment. I have learned from and enjoyed working with many colleagues in the Department of Radiology, chaired by Edmund A. Franken, including Peter Kirchner, Karim Rezai, James Ehrhard, William Yuh, Richard Hichwa, Leonard Watkins, and Laura Ponto. My colleagues and team-members in the MHCRC and Departments of Psychiatry and Speech Pathology have also provided both invaluable contributions and valued friendships: Stephan Arndt, Randy Alliger, Dan O’Leary, Richard Hurtig, Margaret Rogers, Keith Rogers, Victor Swayze, Michael Flaum, Del Miller, Peg Nopoulos, Sanjay Gupta, Ted Cizadlo, Gred Harris, Gregg Cohen, Gene Zeien, Olivia Stibolt, Larry Toothman, Frank Fleming, Mary JKanak, Sally Verhelst, Colleen Peterson, Steve Ziebell, Gary Tyrrell, Dave Elleseon, Bill Hubbard, Carol McNamara, Jane Therme, Kurt Kuebler, Alane Tranel, Kathy Burrows, Kelly Hahn, Melissa Mandrell, Dixie Bixler, Shirley Harland, Marie Samson, Bob Robinson, George Winokur, Bill Coryell, Bruce Pfohl, Ray Crowe, Donald Black, Russ Noyes, Remi Cadoret, and Rafiq Waziri.
Most important of all, I would like to thank the patients, family members, and healthy volunteer subjects who have participated in the research I am describing. Without their generous donation of their time and experience to helping others who suffer, this research would not be possible.