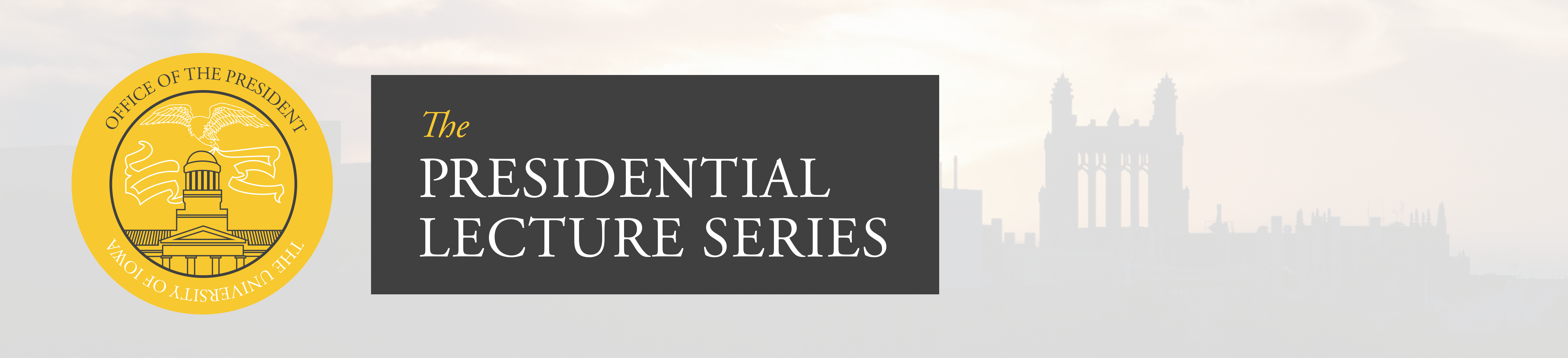
9th Annual Presidential Lecture Series
https://doi.org/10.17077/9hdw-k4km
The Modern Saga of Planetary Exploration
Regent Distinguished Professor
Department of Physics and Astronomy
The University of Iowa
Mr. President, Ladies and Gentlemen:
My professional colleagues and I are fond of calling the past three decades “The Golden Era of Planetary Astronomy.” By extension—a modest one in our view—we are inclined to claim that this era is one of the most significant periods in the history of science. Also, we are much given to mutual congratulations on the good fortune that has enabled us to participate in this great saga of adventure and discovery.
A skeptical observer may note a similar level of professional chauvinism among biologists, physicians, high-energy physicists, and many other specialists. They are entitled to present their own cases. But this is my talk and I propose to discuss modern planetary exploration and to do so with an admixture of subjectivity.
As a young student, I developed a deep admiration for scientists. Among my special heroes were Copernicus, Galileo, Kepler, Newton, Maxwell, and Rutherford. To adopt a term that Tom Wolfe has popularized in another context, these were individuals who were made of the “right stuff.” In fact, I came to the opinion that all other fields of human endeavor (though perhaps very difficult) were intellectually mushy—not possessing the toughmindedness, creativity, and rigor of the physical sciences, and, of course, their working tool mathematics.
I wish to make a public confession here today that this youthful opinion has been shattered many times, particularly by my contacts with colleagues in the many and diverse fields at this University.
But there is something different about science. Students of the human condition tend to have a rather somber if not a gloomy view of the future. By way of contrast, scientists tend to be upbeat and eager to participate in new developments as they unfold on a daily basis. They are inspired by the title of Vannevar Bush’s book Science—The Endless Frontier and they approach their work with a light heart and great expectations. My talk today embodies that point of view.
Also, I will indicate the admixture of science, technology, and, yes, politics which has characterized planetary exploration. I have come to recognize that without appropriate politics there would be very little science.
At this point I have assembled a number of common though disparate assertions. I hasten to remark that these assertions are statements of opinion, not theorems.
- Astronomy is the most ancient and honorable of the physical sciences.
- Astronomy is at the forefront of contemporary intellectual activities.
- Modern astronomy (including planetary exploration and astrophysics) has no conceivable practical value.
- All of the subordinate elements of the solar system—planets and their satellites, asteroids, comets, meteoroids, dust, and gas—are only minor debris left over haphazardly from the formation of a quite mediocre star, the Sun. (This assertion is a nearly verbatim one from a distinguished astrophysicist friend of mine, whose name I have agreed to not mention. But he did smile as he said it.)
- On several occasions I myself have asserted that one could, as a mental exercise, abolish all objects in the Universe except the Sun, the Moon, and the Earth and that most people would never know the difference in everyday life.
- Astronomy is merely one of many applied branches of basic physics.
- The only defensible purpose of publicly supported science is its potential for utilitarian applications.
Each of these assertions has a measure of validity but each one is contestable. They illustrate the well known tendency to exalt and repeat partial truths.
I will now address these assertions in an oblique but, I hope, recognizable way.
One assertion that I consider difficult to contest is that a significant fraction of our population is interested in planetary exploration and considers it “worthwhile.” In the realm of public policy “worthwhileness” may be defined operationally as the level of effort and expenditure that represents a kind of equilibrium between advocates and skeptics.
A special appeal of astronomy is that it has to do with where the Earth came from and when, and where it is going and when. What could be a more fundamental subject? Also astronomy has a grandeur of scale, in both space and time, that gives it a special stature.
I recognize that many other scholarly fields have their places in the full scenario of life. But what about the existence of the Earth, the Sun, the Moon, and all other objects in the Universe? What about the origin of the entire mix of chemical elements that makes life possible? These are matters of astronomy matters—that give profundity to the subject.
Our entire concept of time, both in general and in practice, comes from the daily rotation of the Earth, the monthly orbital motion of the Moon about the Earth, and the annual orbital motion of the Earth around the Sun. This concept is extended by recognition of the orbital motion of the other planets about the Sun.
All forms of life on Earth are astronomically regulated—day and night, the run of the seasons, the rhythm of the tides, and the massive geophysical effects of all of the above. Moreover, no form of life on Earth would be possible without the radiations from our hot, gaseous Sun.
Historically, Copernicus was the most influential person in giving the Earth and its human inhabitants their comeuppance by shifting attention from the geocentric view that the Earth is stationary and the central body of the solar system (and perhaps the Universe) to the view that the Earth is only one of several planets in orbit about the Sun and that it has no claim to astronomical distinction.
Much later, it was Isaac Newton who first recognized, in a somewhat romanticized account, that the force that causes an apple to fall from a tree to the ground is the same force that holds our fast-moving Moon in its orbit about the Earth and the even faster moving planets in their orbits about the Sun.
This force is called Newton’s Universal Law of Gravitation. The adjective “universal” represents our presumption that this law is operative everywhere in the Universe, until convinced to the contrary. The other crucial development in the field of mechanics was Newton’s formulation of his justly celebrated three laws of motion, building on Galileo’s early work. The recognition of these laws of motion also owed much to planetary astronomy.

Mid-twentieth century
The direct prelude to the space age occurred during the period 1946–1957, during which a small cadre of experimentalists conducted investigations through and above the appreciable atmosphere of the Earth with rocket-borne scientific instruments. During the latter portion of this period, our long-held aspiration to extend this work with artificial satellites became a matter for realistic planning. The principles for placing objects in durable orbits around the Earth were thoroughly understood by Newton and explained in his Philosophiae Naturalis Principia Mathematica, published in 1687. But it took some 270 years to develop the practical technology for actually doing so. The most well known elements of such technology were largely the products of military developments—high performance rockets, electronically controlled guidance systems, and radio telemetry. The other crucial but less often mentioned elements were achievements of solid state physicists by the invention and development of the transistor, whose electrical power consumption and weight are far less than those of the previously used vacuum tube, and by the development of efficient solar cells for the conversion of sunlight to electric power. An orbiting satellite or spacecraft would have had exceedingly limited value without these elements.
As part of the planning for the International Geophysical Year 1957–58, a memorable conference occurred in Barcelona, Spain in the summer of 1956. On that occasion we described the U.S. plans for scientific satellites and representatives of the Soviet Union described theirs.
The Soviets were the first to succeed with their Sputnik I in October 1957. But less than four months later the U.S. placed its first satellite Explorer I in orbit. This satellite carried a radiation detector that was conceived and built at the University of Iowa in the basement of the old Physics Building, now called MacLean Hall.
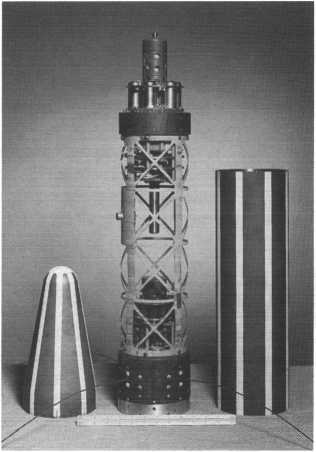
The scientific payload
and its two part protective shield of the first successfully launched American satellite (31January 1958). Note the one-foot ruler for scale. The centrally located cylindrical object about one inch in diameter and six inches long is the Geiger-Mueller tube whose output revealed the existence of the inner radiation belt of the Earth. The radiation detection system was developed and built at the University of Iowa.

William H. Pickering, James A. Van Allen, and Wernher von Braun
hold aloft a full scale model of the Explorer I satellite at a press conference in the Great Hall of the National Academy of Sciences in the early hours of 1 February 1958, following confirmation that Explorer I had completed its first orbit of the Earth.
Explorer I
With Explorer I and its prompt successor Explorer III, we discovered and surveyed the radiation belts of the Earth. This early work spawned a new field of research, later called magnetospheric physics. This subject, in all of its complexity, has engaged the subsequent efforts of a large number of us here and continues to do so, thirty-four years later. We have been joined by literally hundreds of other investigators throughout the world. I have an intimate knowledge of the vigor of this field in my current service as acting editor of the monthly Journal of Geophysical Research—Space Physics, which is devoted primarily to magnetospheric physics. During 1991 under Professor Goertz’ editorship, JGR published 508 highly-technical research papers by 1605 authors, 33% of whom were from thirty foreign countries. The rate of submission of manuscripts continues unabated as I can personally attest.
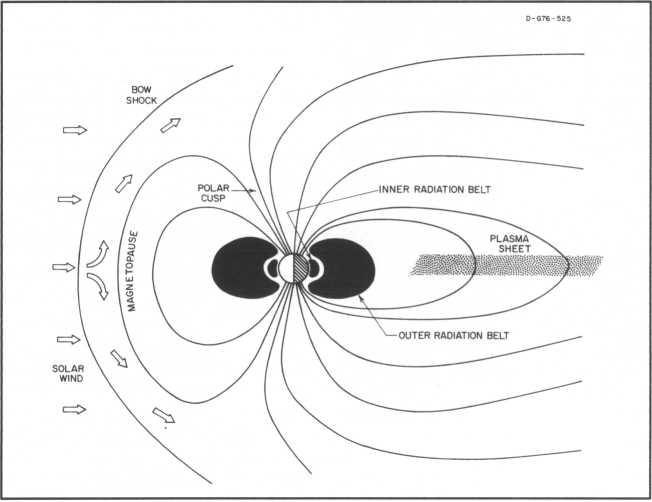

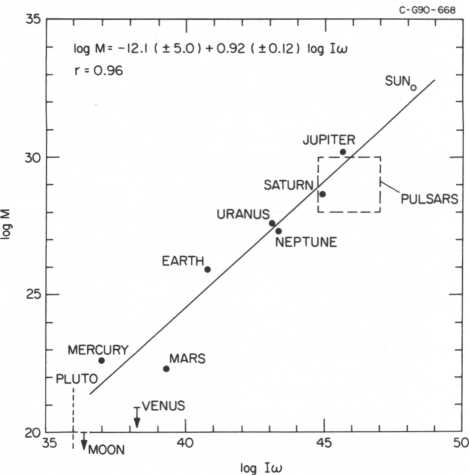
Quasi-military space race
During 1958, the tempo of space flight accelerated dramatically as the quasi-military space race between the U.S. and the U.S.S.R. intensified. Even Congress was stirred to take action and did so with uncharacteristic speed. Under the spirited leadership of Lyndon Johnson in the Senate and John McCormick in the House, the peaceful uses of space technology were declared to be a national purpose. The relevant legislation was called the Space Act of 1958. It was signed by President Eisenhower in October 1958 and became an element of national policy. The first of the eight listed objectives of such work was declared to be
The expansion of human knowledge of phenomena in the atmosphere and space.
It is under the authorization of this one thin sentence that all of the U.S. scientific work in space has prospered for the past 34 years.
As an aside, I note that no one of the declared objectives in the Space Act mentions the space flight of human crews. Advocates of such activities adopt the view that they are authorized by implication under an objective that includes the transport of “living organisms through space” or under another objective that mentions advances in aeronautics.
A noteworthy element in planning scientific work in space was the 1962 summer study of the Space Science Board of the National Academy of Sciences. This six-weeks’ workshop under my chairmanship was conducted on this campus in spacious quarters in the then not-quite-completed University Library by the courtesy of Professor Dunlap, the University of Iowa librarian. Our final report entitled “A Review of Space Research” had a durable influence on subsequent undertakings and remains, even today, a valuable reference document.
Later that year, but not as a direct result of this workshop, the U.S. conducted the first ever exploration of another planet, Venus, with the spacecraft called Mariner II. The first successful mission to Mars was by Mariner IV in 1967. Both of these pioneering missions carried University of Iowa instruments.
The pace of planetary missions soon accelerated with comparable efforts by the U.S. and the USSR.
Early missions to extraterrestrial objects were devoted exclusively to the Moon, Mars, and Venus.
The principal reasons for this emphasis were (a) that these objects were relatively easy to reach by virtue of their proximity to the Earth; (b) that, for the same reason, previous knowledge of them was sufficient to facilitate the formulation of well-posed objectives; and (c) that there was an overarching wish to search for any evidence of extraterrestrial life, past or present, on the Moon or, more likely it was thought, on Mars. I supported these objectives but my own special interest was in the great outer gaseous planets Jupiter, Saturn, Uranus, and Neptune because of their totally different physical nature than that of the inner terrestrial or solid planets (Earth, Venus, Mars, and Mercury) and the probability of much more exotic phenomena.
In the late 1960s I made such a nuisance of myself as a member of both the Space Science Board and the Lunar and Planetary Missions Board that I was appointed chairman of two different groups to work with NASA in advocating and planning missions to the outer planets. The first fruits of these efforts were approval and funding of two missions to Jupiter, later called Pioneer 10 and Pioneer 11, both carrying Iowa instruments. Pioneer 10 was launched in 1972. It made the first ever encounter with Jupiter twenty months later with spectacular success and has continued on a solar system escape trajectory. At the present time it continues to operate well and to yield important observations in the outer solar system. It is the most remote manmade object in the Universe, now nearly five billion miles away. A month from today [February 2, 1992] we will celebrate its 20th in-flight birthday. Its companion spacecraft, Pioneer 11, now nearly 19 years old in flight, made the second encounter with Jupiter and, far exceeding its originally stated objectives, made the first ever encounter with Saturn in 1979, again with striking success. It is also still operating in the outer solar system, as it gradually escapes therefrom.


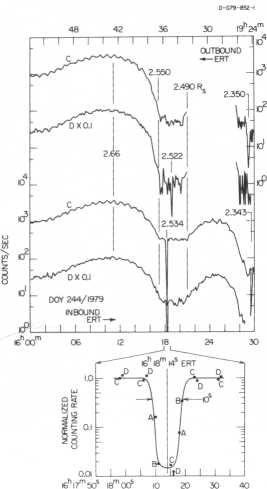
Voyager 1 & 2
Two successor missions to the outer planets, Voyager 1 and Voyager 2, were launched in the summer of 1977. Each carried a much more sophisticated array of scientific instruments than that on the Pioneers, including an Iowa instrument of high quality. Each has yielded many important advances in knowledge of Jupiter and Saturn. Voyager 2’s encounter with Saturn was designed so that it subsequently made the first ever encounters with Uranus in 1986 and Neptune in 1989—both historic achievements.
The composite results from these four spacecraft have yielded dozens of detailed papers and a series of research monographs, which represent a truly revolutionary advance in planetary astronomy.


Ulysses and Galileo
Two other outer planet missions are currently in progress.
The European Space Agency’s spacecraft Ulysses will fly by Jupiter on the 8th of this month and continue on an exploratory mission to high solar latitudes for the first time during the subsequent four years.
The other current mission in this category is Galileo, en route to Jupiter via a very circuitous path—by Venus, by the Earth, by the asteroid Gaspra, and by the Earth again (next December). Two of the nine scientific instruments on Galileo were developed by Iowa groups led by Professor Frank and Professor Gurnett. Their instruments are performing well but efforts to deploy or unfold the umbrella-like high-gain antenna on the spacecraft have thus far been unsuccessful. Despite this handicap, it remains feasible to fulfill one of the important objectives of this mission, namely the delivery of a deep entry probe into Jupiter’s atmosphere to make the first in site survey of its composition, structure, and other basic properties. It is also feasible to inject the mother spacecraft into the intended orbit about Jupiter. There is a certain level of optimism that efforts to unfold the high-gain antenna will eventually succeed. If so, the planned radio telemetry rate of some 120,000 bits per second will be available and a rich variety of new results on the planet itself and on its four Galilean satellites can be anticipated beginning in late 1995. If not, the results from orbit will be of interest but there will be a dramatic reduction in detail because of the very limited telemetry rate provided by the low-gain antenna.
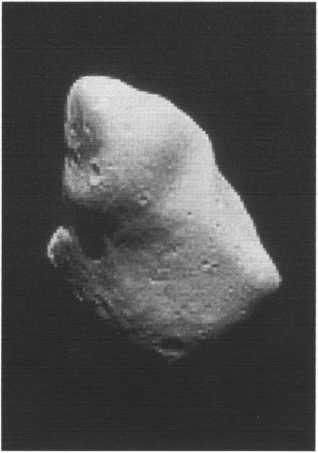
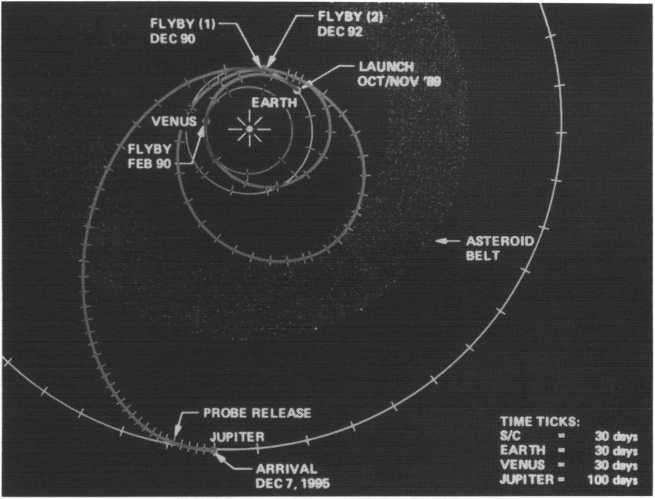
Viking, Pioneer, and Magellan
Meanwhile, there have been a large number of missions both U.S. and Soviet to the Moon, Venus, Mars, and Mercury.
Noteworthy among them were two Viking missions to Mars, each of which included one lander and one orbiter. The landers were designed specifically to search for the existence of any form of life by detailed physical and biological assays of the surface material. The relevant equipment worked well and yielded impressively negative results. But, in fairness, it should be added that there are still lines of thought, which suggest a lack of broad conclusiveness on this important matter.
There are two currently active planetary missions in the inner solar system. The two-part Pioneer Venus mission conducted observations with sondes lowered by balloons through the incredibly dense, hot atmosphere of Venus in 1978; and the orbiting spacecraft of the mission has been operating continuously since that date and has been the source of a steady flow of important new results.
The Magellan spacecraft, in orbit about Venus since 1990, is using a high-performance radar-altimeter to produce topographic maps of the surface of Venus having the unprecedented resolution of about 100 meters.



A Final Note...
Future U.S. planetary missions, which are authorized, funded, and under development include the Mars Observer, the Cassini mission to Saturn and its largest satellite Titan, and a comet rendezvous asteroid flyby mission called CRAF.
A resumption of manned missions to the Moon and the conduct of one or more manned missions to Mars are widely discussed. But at the present time, they hover in the arena of futurism and presidential rhetoric and are not realistic prospects during the present century.