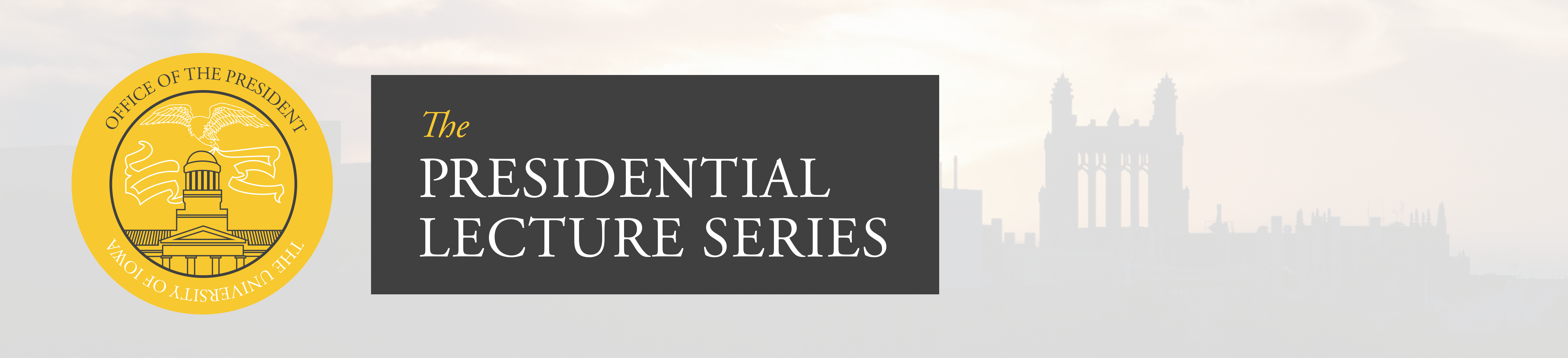
2nd Annual Presidential Lecture Series
https://doi.org/10.17077/k8x1-gvpg
What’s in a Brain That Ink May Character?
Professor of Neurology
The University of Iowa
College of Medicine
This lecture is dedicated to Norman Geschwind, Maurice W. Van Allen, and Arthur L. Benton
Prefatory remarks
James O. Freedman
President
The University of Iowa
Vice-President Remington, Dean Eckstein, Ladies, and Gentlemen:
We are about to share one of the most exciting experiences of the academic year. Dr. Antonio R. Damasio will continue one of our newest traditions at The University of Iowa as he delivers the second in the annual series of Presidential Lectures.
The Presidential Lecture series is a celebration of the life of the mind. It celebrates the joy of research, the excitement of intellectual interchange, and the disciplined pursuit of ideas that matter.
It celebrates the vital traditions of scholarship through which senior scholars inspire younger scholars and students at the same time that they are making new contributions to that living body of knowledge from which all of us derive strength.
We are honored that the 1985 Presidential Lecture is to be delivered by Dr. Antonio R. Damasio, professor of neurology and chief of the Division of Behavioral Neurology of the College of Medicine, where he is also director of the Alzheimer’s Disease Research Center.
Professor Damasio is internationally recognized for his teaching and research in the new field of behavioral neurology. Under his leadership, The University of Iowa has become one of the pioneering centers of research into the relationship between brain function and behavior.
Professor Damasio received both the Doctor of Medicine and the Doctor of Philosophy degrees from the University of Lisbon. He joined The University of Iowa faculty in 1976 and is the author of more than 140 publications on anatomical aspects of higher brain function, parkinsonism, and dementia.
Professor Damasio’s research focuses on the cerebral basis of such processes as vision, language, and memory and on the application of the scientific advances in those areas to the diagnosis and treatment of such diseases as Alzheimer’s.
His lecture will highlight recent developments in the understanding of how brain function relates to memory and language and how those developments influence the management of Alzheimer’s and related diseases.
The title of his lecture—“What’s in a Brain That Ink May Character?”—is taken from the first line of Shakespeare’s Sonnet 108. Dr. Damasio will use “ink” to refer to the sophisticated imaging and staining techniques that have made modern brain research possible.
Knowing that Professor Damasio was likely to emphasize that the study of the brain requires the perspectives of both the scientist and the humanist, I thought it wise to prepare for this occasion by returning, as he had, to Shakespeare’s sonnets.
I wanted to see whether Shakespeare had dropped any clues on how the brain functions in “remembrance of things past.”
Sonnet 108, from which Dr. Damasio has drawn the title of his lecture, did not refer to the subject after the first line. But in the opening lines of Sonnet 122, I found that Shakespeare had indeed recognized the neurological basis of his memories of his friend.
That gift, thy tables, are within my brain
Full character’d with lasting memory
Which shall above that idle rank remain
Beyond all date, even to eternity;
Or at the least, so long as brain and heart
Have faculty by nature to subsist;
Till each to razed oblivion yield his part
Of thee, thy record never can be missed.
And now we turn to the scientific elaboration of Shakespeare’s momentous discovery. I am delighted to introduce Professor Antonio R. Damasio, who will lecture on “What’s in a Brain That Ink May Character?”
Opening Statement
President Freedman, Vice-President Remington, Dean Eckstein, Colleagues, Ladies, and Gentlemen:
It is difficult to imagine progress without specialization in science, technology, or artistic endeavor. And yet, this necessary specialization threatens the quality of intellectual life and, left unchecked, is likely to erode the fabric of universities, transforming them into a collection of teaching and research centers of greater or lesser excellence. President Freedman’s academic program indicated, early on, his preoccupation with this growing problem, his commitment to the promotion of a meeting ground for the men and women who provide academic progress in an atmosphere in which universitas would maintain its meaning. So it did not come as a surprise to hear of the institution of the Presidential Lectures but it was certainly a surprise to be invited to deliver one of the first. A surprise, a privilege, and a heavy responsibility: for this is not a lecture for neuroscientists, my stock in trade, but an address to colleagues of other disciplines, who may be unfamiliar with the vocabulary of the neurological sciences. My only hope for some success resides with the interest that I know we all share, in the elusive connection between brain and mind.
Introduction
The subject of this lecture is the relation between brain and behavior, more specifically, between the human brain and higher behavior. That one and the other are associated is not new. Shakespeare certainly knew of the connection when he wrote the richly ambiguous title of my lecture, and he used that knowledge frequently. There are at least a dozen references to brain in Hamlet alone, just as many in Othello. In these and other plays such references cover a large range of psychological states, as when Shakespeare has Queen Gertrude say of Hamlet “And, in this brainish apprehension, kills the unseen good old man,” and thus poor Polonius is dispatched for the evening; or when King Henry VI talks about the “madness that rules in brainsick men.” Shakespeare also used the connection for humorous effect as in Cymbeline. Says the First Lord in act 1: “Sir, as I told you always, her beauty and her brain go not together.”
But the intricate details of the brain/behavior relationship, the manner with which the brain subserves the fine faculties of, say, language, memory, or problem solving, has largely remained a mystery. As recently as one century ago, there were hardly any testable hypotheses regarding that relationship. In our century, as varied evidence has accumulated, obtained with different methods and informed by different theories, the issue has often become more clouded than clear, the ebb and flow of findings alternatively suggesting that the riddle could either be solved or would remain untouched. In the past two decades, however, it has become obvious that the problem can and will be solved. You may ask why now? And the answer is that the conglomerate of disciplines we collectively designate as the neurosciences has been developing explosively, especially in the past ten years. The result has been the opening of several new fronts in the attack of the general issue of nervous system operation. Every level of the nervous system is under scrutiny in small organisms, rodents, nonhuman primates and humans. Riches are found and stockpiled regularly. It would take me several hours to give you a catalog of recent, worthy achievements. The large annual meeting of the Society of Neuroscience no longer can do them justice, no journal of neurological sciences even tries, and journals such as Science have given up covering, at any one time, anything but narrow albeit important angles of this vast panorama. What I can do, nonetheless, is tell you about some of the developments I consider most pertinent. For instance
- The current interest in growth factors of neurons (nerve cells)
and glial cells (cells that form the structural and functional tissue support
for neurons) is likely to let us understand how, during maturation, the
developing nerve cells find the inexorable path to their appropriate match,
amid the maelstrom constituted by millions of others, how they recognize their
social synaptic contacts, how they say hello, fascinate each other, and enter a
synaptic relationship till death does them part. This may one day tell us how
vastly different nerve cells with different role assignments come to be wired
in a given network. It may also tell us why, in some of us, there are
miswirings. Projecting further into the future, we may come to know the genetic
machinery that controls such operations and correct the disturbed ones. The
discovery of an abnormal gene in chromosome 4 of patients with Huntington’s
disease tells us this is feasible, for a few neurological diseases with a
strong inheritance pattern, although it is not possible to predict when the
good fortune that surrounded that discovery will strike again.
Forty years ago, Roger Sperry was preoccupied with this topic for which he posited the phenomenon of chemotaxis. Now, the providers of chemotaxis are being identified. Some are likely to be hormones, in the broad sense of the term, generated within nerve cells themselves or available in their environment. As the story unfolds, the brain may actually come to be seen, in certain respects, as a giant endocrine system and this possibility, together with the relationship between endocrine and immunological systems, looms large in the future of our field. - Molecular neurobiology techniques are bringing us other developments. For instance, the structure of the nicotinic acetylcholine receptor has now been modeled; and it is even possible to predict the intimate mechanism of the opening of its sodium channels, an important step in understanding mechanisms of cell-to-cell transmission of information. Another example: By making antibodies to antigens in the membranes of specific nerve cells, it is now possible to map different cell types in the nervous system.
- Using techniques of immunohystochemistry in experimental animals, it is possible to map out the storage sites, within neurons, of neurotransmitters and neuromodulators, a variety of substances (generally amines, amino acids, or peptides) concerned with transmission of information from nerve cell to nerve cell. Some of those substances, as the investigations of Stanley Kater at The University of Iowa indicate, may also play a role in the self-organizing development of neurons and of their connections.
- On the other hand, novel tracer substances (for instance, horseradish peroxidase, radiolabeled amino acids) can be injected in the nervous tissue of experimental animals, be taken up by the nerve cell body or by its ending, and move physiologically up or down the axon, staining the cells visibly under the microscope, permitting the mapping of neuron interconnectivity in a way that the father of cellular neuroanatomy, Santiago de Ramon y Cajal, would not have dreamed of; and at the level of individual nerve cells, and also in experimental animals, it is now possible, with fine techniques, to study the behavior of a single cellular unit, by reading out its electrophysiological responses as it is variably stimulated.
- Turning to the larger systems, we now have the ability to look
simultaneously at different components of the nervous system and surprise them
in action while part of a concerted network. We can do this not only in animals
but in man also, and we are now able not only to look at the nervous system disturbed
by one area of damage and failure, but also at the intact system as a whole, as
it operates normally. We can sample (a) electrical reflections of its activity
(with fine electroencephalographic techniques), spontaneous or evoked, or (b)
the differential emission of radioactive particles (single photons or
positrons) from differently activated areas (in the techniques of SPET and PET)
or the (c) differential absorption of X-rays in different brain regions (as in
X-ray computerized tomography, known as CT). Finally, even the different radio
frequencies that different brain areas can transmit when subject to powerful
magnetic fields can be detected, in the technique of magnetic resonance imaging
(MRI, also known as NMR). With the help of tomographic devices, appropriate
algorithms, and fast operating computers, we can reconstruct these events and
map their relative position in the three-dimensional universe occupied by brain
matter.
It has always been possible to place lesions (i.e., circumscribed areas of tissue damage) in the brain of animals and design experiments after those lesions render their effects. But with state-of-the-art imaging technology, it is now possible, under conditions of careful planning, to do precisely the same with the lesions that nature inflicts on human beings, and thus make neurological disease pay a dividend in the form of unique research data. Furthermore, it is possible to model the networks to which the lesions belong and simulate their operation for the highest and most complex cognitive processes. Such models, in turn, lead us to the prediction of function of certain components, or to fruitful hypotheses regarding the possible areas of failure. They may, in the future, permit us to transplant certain lower level components of the brain so as to substitute healthy neurons where diseased ones no longer work.
This may all be impressive, but the challenge is, naturally, to reflect on the discoveries and shape them in a coherent explanatory picture of the brain of man, its function, and its diseases. I am sure you realize that there is considerable debate regarding which of the newly available approaches is more pertinent to the problems of behavioral neuroscience; which approach will hold the key to the secret codes of higher brain function. When partisan argument dies down, the answer to such imperfect questions is unequivocal: probably several of these methods, as needed and as applicable to the specific issue at hand. There is not one hint of compromise in this answer either.
Take, for instance, any of the central issues of brain and higher behavior—the physiology of language or of memory or of vision. Should one approach them from the top down or from below up? Do we start with the macro systems above, from a general angle, and work our way to cell assemblies and molecules, or do we start below, from a piecemeal stand, in hope of finding the system’s underbelly? I was caught in such a crossfire in a conference on the philosophy of science, a few years ago, and got out of it by stating that my choice was from midships, up and down. I meant it. The understanding of complex brain functions must come from the effective deciphering of the codes of each pertinent level and the gleaning of the multilevel integration of those codes. It is important to know how a nerve cell circuit may act, at synaptic levels, to hold down a memory, and to learn about the participation of different mediators in such a process. But such knowledge tells us about a restricted aspect of the complex operation of memory, and it is not possible at all to guess the organization of the high level by merely multiplying what is known about a cell assembly by whatever number of cell assemblies may comprise the entire system. For one simple reason: The brain is not a single organ as we were told in school. It is a gigantic system of systems, each of which does contain organs. The systems have different functional roles and different ranks in the overall hierarchy. The ensemble is easily the most elaborate piece of biological machinery in the universe and the pinnacle of the elaboration is the human brain. Knowledge of the physiology of a cell column within a part of a given association cortex tells us little about the operation of other cortical or subcortical units participating in a given functional network, and tells us absolutely nothing about the overall organization and regulation of that network. It is important to realize that what permits the higher behaviors we want to understand is the operation of the network itself, the actions it performs, the way it manipulates information. What we know about, when we learn about the growth pattern of nerve cells or about the transmitters they use for certain types of firing, has to do with the condition of the network, with the development and physical properties of constituent units, not with the operation of the network. The metaphors of hardware and software are too crude and only apply in part but they capture part of the flavor of this distinction. The key to the understanding of the biology of higher behavior resides, in our view, with cognitive modeling from the top down and with the introduction of as many constraints as we can discover, by appropriate anatomical and physiological probing, at all pertinent subsequent levels. In time the strategy will come to encompass the whole system of systems.
A related question has to do with the best source of data for studies of higher brain and behavior. Does research in humans produce valid findings, some may ask? Here, too, the answer comes from the specific problem we need to solve, the available theories and methods. Such questions have a way of sorting themselves out. To give you an example: If we want to know, and we do, how the human brain develops its prodigious capacity to produce and understand the syntactical structure of language and how it is capable of automatically selecting different lexical items to compose in words the substance of a nonverbal fragment of thinking, we would be ill advised to conduct experiments in dogs or monkeys. Besides, the technology that permits the experimental study of such problems in normal or diseased human beings has finally become available. And yet, it would be unfortunate to overlook the potential for elucidating aspects of those strictly human problems in well-devised animal experiments; it would be a loss not to allow the substance of experiments performed in smaller organisms or lower neural levels to permeate one’s model for the elucidation of the human condition. For instance, we are currently studying the neuronal connections of the auditory cortices of the monkey, well aware that monkeys write no poetry and do not care for the spoken word, but convinced that the basic components that underlie auditory learning in the monkey must have a considerable commonality with those of man.
This brings me to my program for today, which is to illustrate some developments in brain and behavior research that my colleagues and I have been concerned with. In the examples I will discuss, you will sense four underlying themes.
- That the adult human brain honors a principle of functional modularity for higher cognitive networks, as demonstrated by consistent associations between certain behaviors and certain anatomical structures. This modularity holds not only for the sensory motor systems and language—for which a high degree of patterned, hardwired structure is apparent—but also, to some extent, for supramodal central processors of information. The principle is weakened for the lower levels of the nervous system and for lower species in which plasticity is likely to be larger.
- That the results contribute to our understanding of both human higher brain function and neurological disease.
- That such results could not have been obtained in experimental animals, although animal work elucidates some of the questions they pose.
- That the results depend on powerful anatomical and radiological images, the metaphorical ink which I alluded to in the title.
Brain and Language
Our study of the relation between language processing and the brain has aimed at the large scale mapping of such functions as reading, writing, speaking, and comprehension of speech, in relation to syntactic structure and lexical choice. In general, the design is one in which the investigators concerned with anatomical analysis are blinded to experimental behavioral data and those concerned with behavioral study have no knowledge of anatomical findings when they conduct their assessments; in which subjects are entered on the basis of either a type of behavioral disorder or a type of anatomical lesion, with the analysis of each performed independently; in which, ultimately, we proceed to a double regression in search of the match of the two sets of data. There are multiple controls, not only normal subjects matched for age, sex, and education, but also subjects with different disorders and different anatomical loci of lesion, so that overlaps or dissociations may be detected. All the subjects with brain lesions have one single type of disease process, cerebrovascular disease, what is commonly known as stroke.
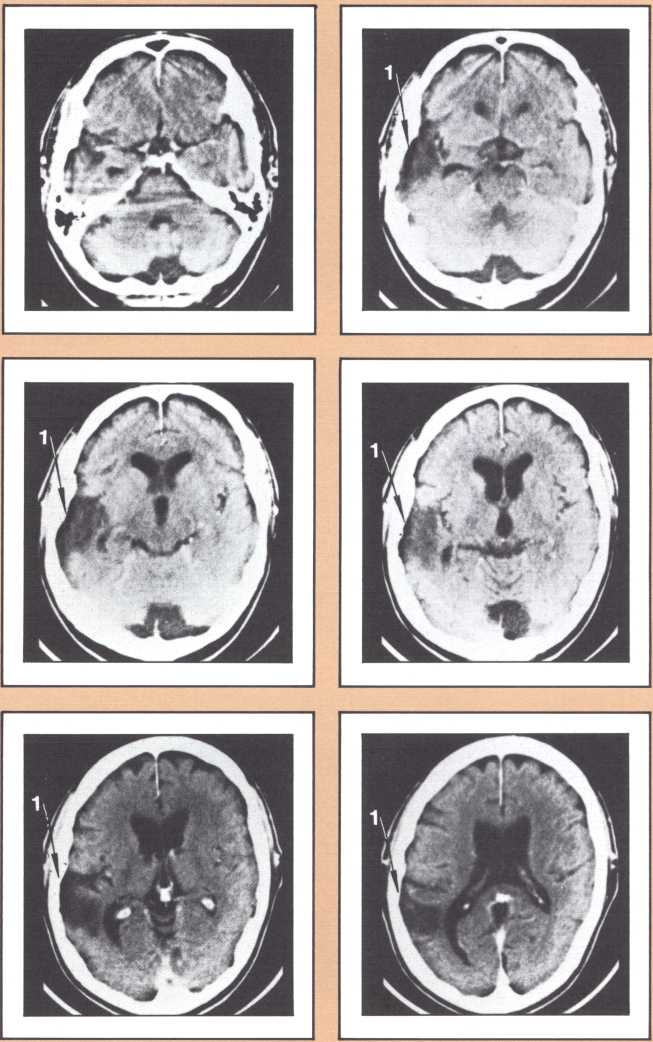
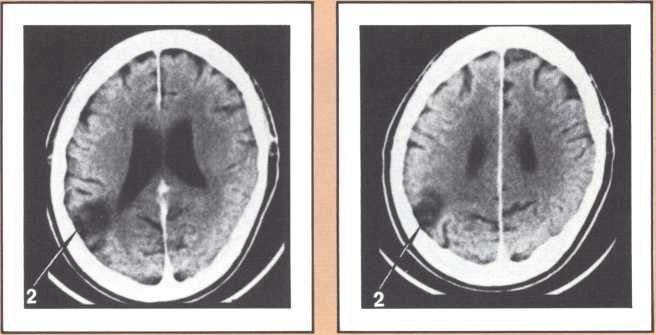
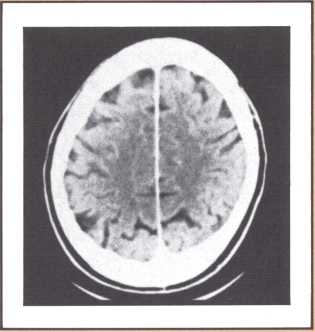
The results obtained in our laboratory over the past ten years strongly suggest that the brain subserves some of these language functions and carries out some grammatical operations with different neural systems. For instance, take the example of this particular subject studied by a team that included Paul Eslinger, Hanna Damasio, and Harold Adams from our unit and Steven Cornell and Val Dunn from the Department of Radiology. As indicated by the X-ray computerized tomography scan, this subject sustained damage to a restricted area of the auditory association cortex in the left hemisphere (figure 1). The exact position of the lesion was detailed by a painstaking process of charting in appropriate brain templates, by Hanna Damasio. Her charting was based on a complex system of landmarks and image transposition, not unlike the work of a cartographer preparing a map (figure 2). This subject’s ability to perform the lexical choice necessary to communicate his thinking process in verbal terms was grossly disturbed. You may try to picture it as an inability to access a dictionary in your search for the proper translation of a given noun or verb, except that the translation that we are considering in this person was between a nonverbal, internal representation and its verbal counterpart. Yet, this person had no problem in assembling the grammatical structure of the sentence whose referential lexicon he was unable to implement, or in choosing the functor (closed-class) words that impart additional meaning to an utterance. Here we have an astonishing dissociation. The ability to achieve correct word order in a sentence, respecting, for instance, the legitimate positions of verb and object, to select and place conjunctions and prepositions, and even to append the concordant verb endings, was maintained. The ability to evoke lexical items, however, was either blocked or highly distorted, giving rise to inappropriate word usage, what we know in neurological terminology as a paraphasia. A patient in these circumstances, incidentally, is known as an “aphasic.” When this dissociation between open-class lexical and syntactical processing takes place and is accompanied by normal rate of speech production and defects in auditory comprehension and repetition, it is known as the syndrome of Wernicke’s aphasia. It must be understood that characterizing this patient’s linguistic difficulty was important to help diagnose his neurological condition and to plan strategies for his recovery so that he could cope with such a defect. But, as I pointed out earlier, we are trying to make the disease pay dividends by allowing us to study what the brain is and is not able to perform, linguistically and cognitively, once it has been insulted in this fashion. By analyzing multiple cases, it enables us to decide how the diverse positioning of lesions in this vicinity will affect the linguistic processing in a different fashion and by doing so allow us to construct models of function and dysfunction that can be subject to appropriate test later on.
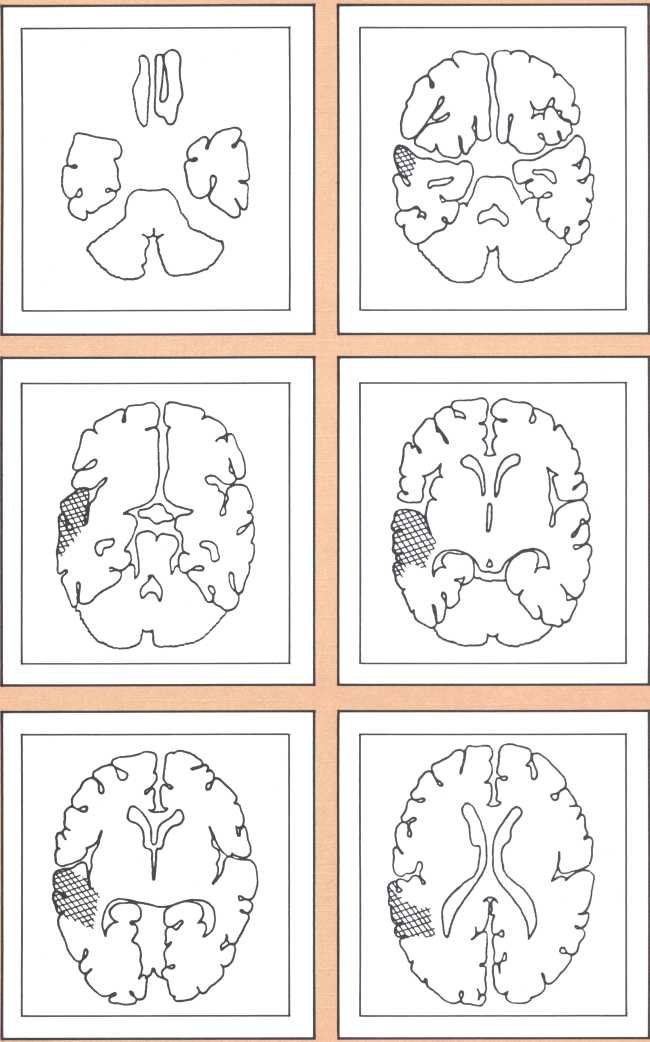
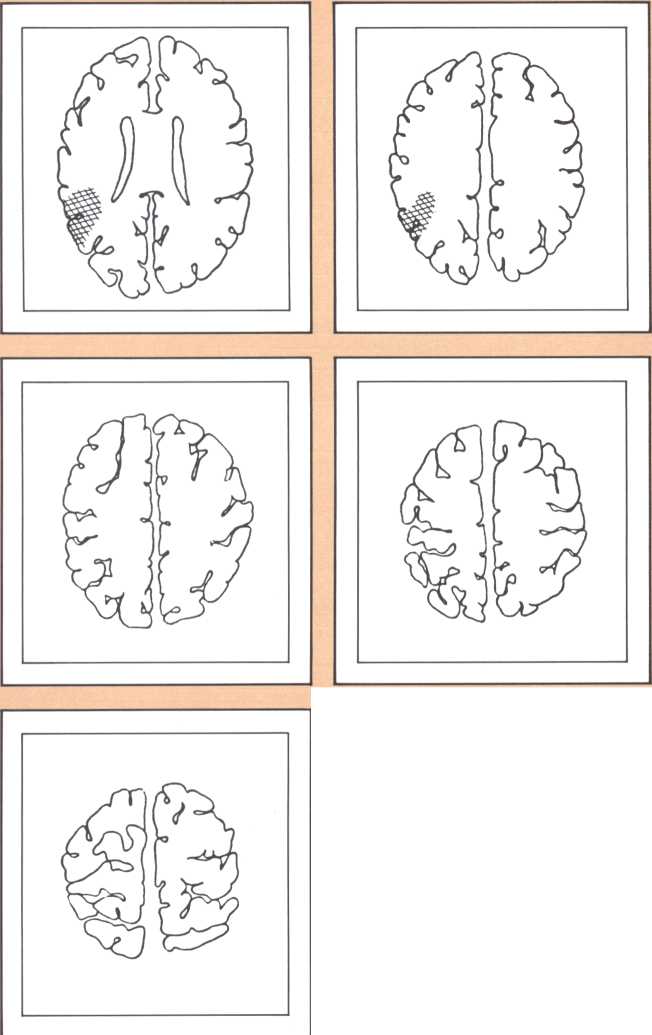
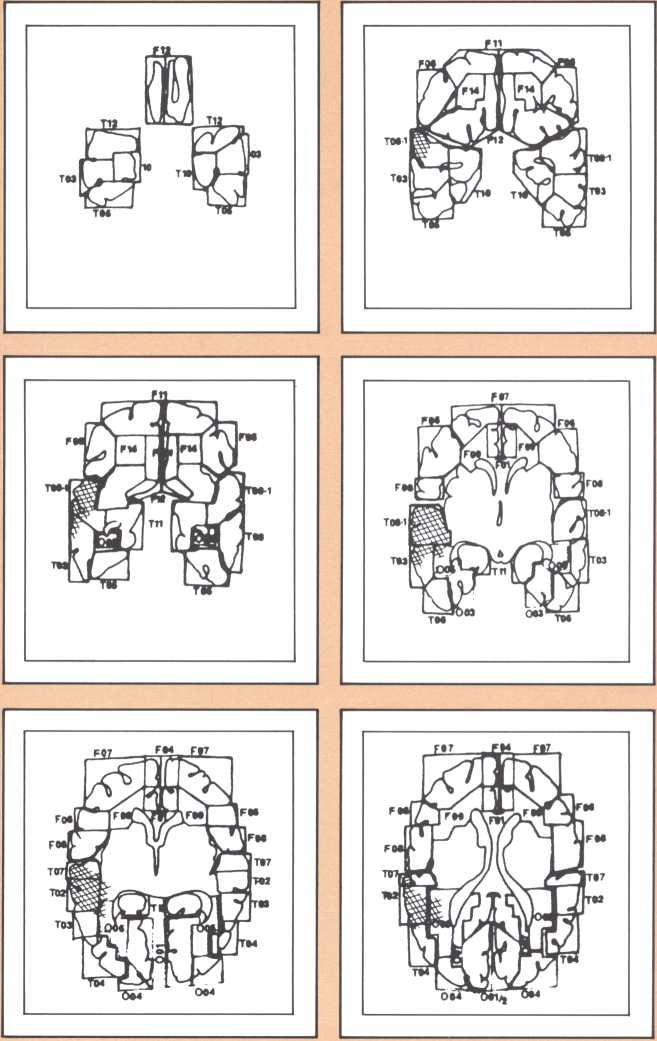
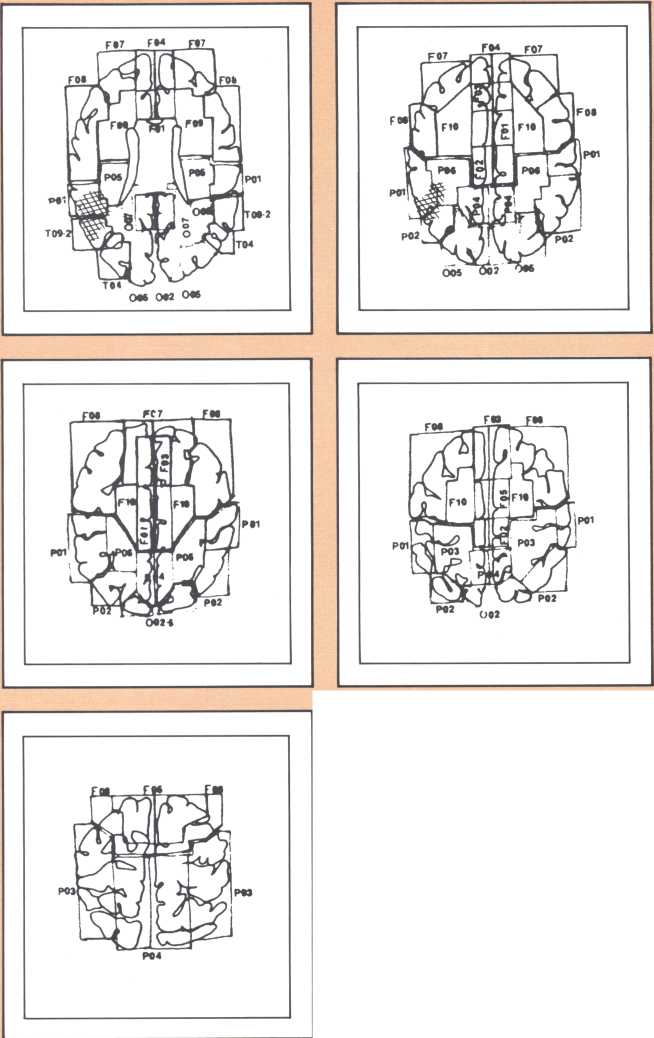
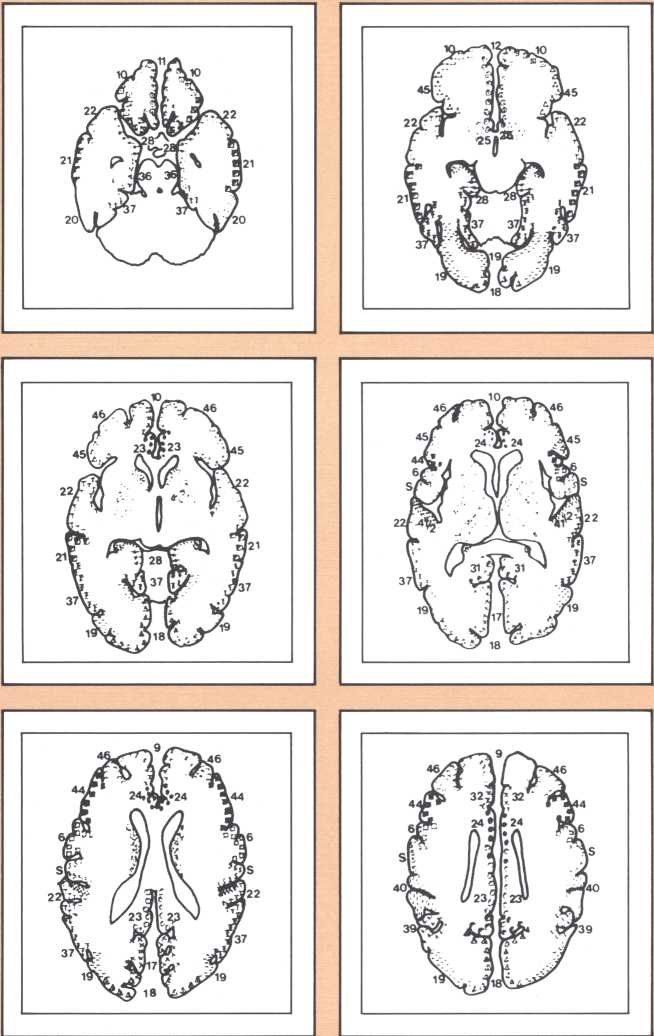
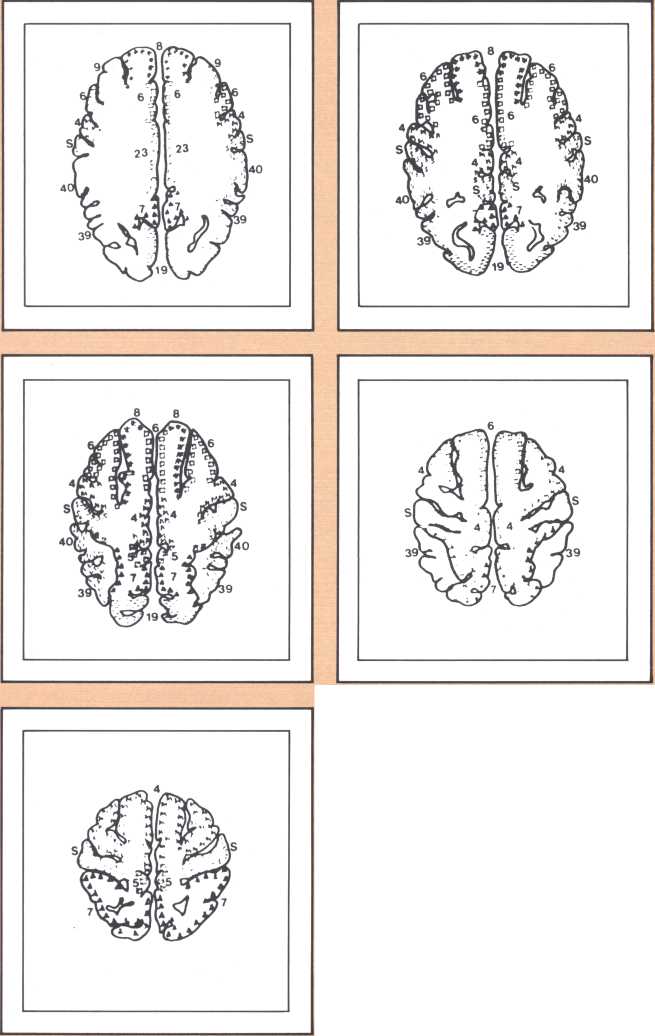
The following image provides a comparable example, studied with magnetic resonance, the newest and most detailed type of imaging of the brain (figure 3). It permits a full view of the living brain with the anatomical detail that until just a couple of years ago was only accessible in post mortem studies, at the autopsy table.
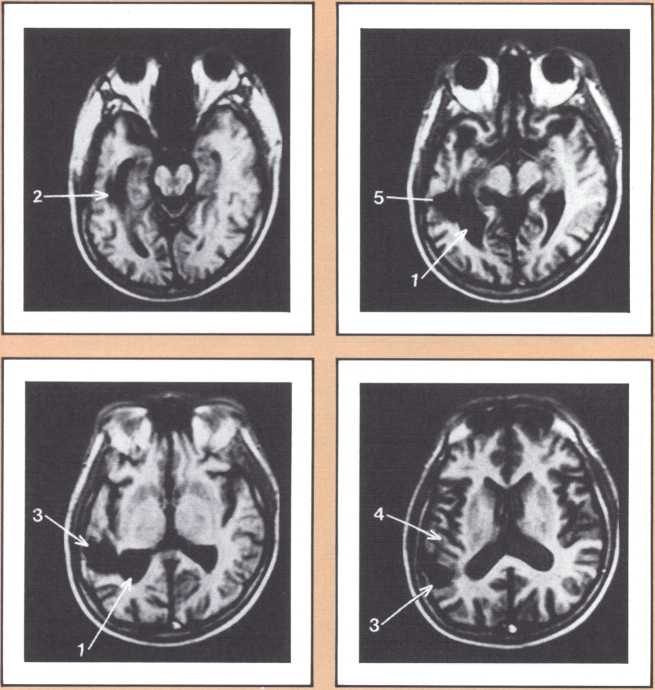
The subject whose brain you are looking at now had a radically different type of language impairment. Her ability to evoke the proper lexical choice was generally maintained. What she had become unable to do, unlike the previous subjects, was to organize the syntactical structure of her sentence. So, even when she selected the appropriate nouns or verbs to express her nonverbal meanings, those words failed to be linked by the functor elements of language (closed class), such as conjunctions and prepositions, all those words that have no external reference but that provide the relational fabric of language. These specific characteristics, along with the presence of a repetition defect and a right hemiparesis, qualified this subject for the diagnosis of Broca’s aphasia.
The subjects I have mentioned are paradigmatic of two aphasia syndromes. There are numerous other syndromes that affect communication and cognition in distinctive manners. The important message is that there is a clear relationship between the linguistic and cognitive impairments and the exact position of the lesions that brings them about, that is, different lesions, by involving different brain units, cause different functional impairments. After analysis, with the methods that I have outlined, of numerous subjects in our laboratory and in others, it is possible to say that the effects of these lesions are generally predictable and that the prediction holds for a large segment of the human adult population that has “standard organization” for language in the left, so-called dominant, hemisphere of the brain. For such a population, the reversal of these anatomical-behavioral associations that I have described has never been observed. For the nonstandard population there are partial exceptions to the rule. They occur largely in subjects who have their neuroanatomical endowment for language in the opposite hemisphere or distributed in varying proportions between the two hemispheres. Most, though not all, of such subjects are left-handed. It is important to note that many left-handers appear to have entirely standard brain organization for language.
The current challenge is to perfect the relationship to understand the multiple components of the network that relate to a given linguistic process, to guess which alternative networks perform the same or equivalent roles, to connect more and more subtle aspects of the behavior with more and more circumscribed areas of nervous tissue.
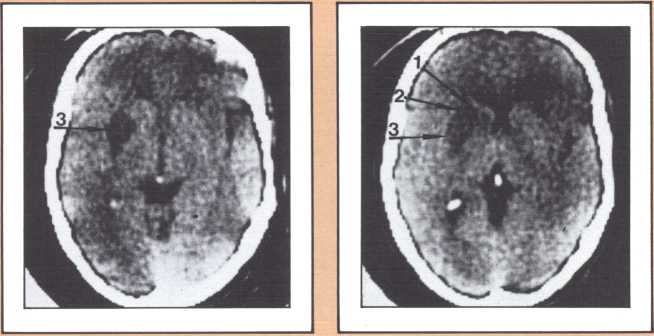
The blind analysis of these disturbances allowed us, in the late seventies, to detect a novel, and until then unpredicted, association between language impairments and lesions of the gray, deep structures of the brain, known as the basal ganglia and the thalamus. Subjects with damage to the head of the left caudate nucleus and to the anterior limb of the left internal capsule, develop an impairment of language processing different from those I have just described (figure 4). Lesions of the basal ganglia away from this locus or of the nondominant basal ganglia fail to cause aphasia. This finding has been replicated now by several laboratories in this country and in Europe, and we are engaged in understanding the mechanism through which such lesions cause a language impairment. Physiological recording of the activity of these neurons indicates that they are heavily involved in a form of motor programming, but that, in addition, they probably play a role in perception as well in the monitoring of ongoing perceptual activity and in the modification of the organism in relation to ongoing processing. My colleague Gary Van Hoesen has begun to elucidate the anatomical structure of these areas in nonhuman primates. Together with Hanna Damasio’s imaging studies, the findings show that the primary locus of lesion in humans corresponds to an area that receives a maximal amount of auditory projections. Our current hypothesis is that lesions in this area interfere with the monitoring of auditory processing in humans, in addition to modifying several aspects of the motor programming necessary for language. Another form of language disturbance has been seen in relation to anterior left thalamic nuclei, as indicated in a study by Neill Graff-Radford in our unit.
The dedication of the left hemisphere to language phenomena is currently attributed to a powerful asymmetry of language-related structures in the human brain. In a disarmingly simple study published in Science in 1968, Norman Geschwind studied 100 consecutive normal brains of human adults at autopsy and found that the planum temporale, the area of auditory association cortices located behind the primary auditory field (or Heschl gyrus), was several-fold larger in the left hemisphere than in the right, in about 70 percent of the brains. A part of his sample had either equal plana on the left and right, or actually a larger planum on the right. Later it was found that lower nonhuman primates, such as the rhesus monkey, do not have that asymmetry, and that some higher apes have it in a minor form. Considering that no other such anatomical asymmetries have been noted in human brains and taking into account that these asymmetries have now been replicated in numerous laboratories, it is likely that they do reflect a different anatomical endowment for language and that the clear majority of right-handed individuals and a part of left-handed ones possess this anatomical disposition. This feature is accompanied by an anatomical asymmetry of the form of the parietal operculum, which can be detected in the anterior-posterior view of bilateral angiograms and in the fine cytoarchitecture of this area in humans. (Cytoarchitecture is the study of the spatial and connectional relationship of different types of neurons in different brain areas.) It is of interest to know that human fetuses already show this asymmetry about the sixteenth week, a good indication that it is genetically engendered; and that there is preliminary evidence from the endocasts of anthropologically studied skulls that this asymmetry may have been present in man for at least 30,000 years. Here is a picture of the endocasts from the La Chappelle aux Saints, in which the different direction of the sylvian fissure is clearly indicated. There are numerous indirect ways of predicting the changeover of endowment from the left to the right hemisphere in relation to language and one of the finest is with emission tomography.
The emission tomography scan in figure 5A is that of a young and intelligent woman who suffered from intractable seizures (epileptic fits refractory to any appropriate medication). It was possible to treat her seizures effectively by removing surgically her right temporal lobe, provided we could confirm that the seizures were arising exclusively from there, and that her right temporal lobe was not involved with language processing. For this reason a detailed and multifaceted study of her brain function was undertaken at The University of Iowa Hospitals and Clinics by members of our unit and by her neurosurgeon, John Van Gilder. I will cut a long story short by telling you that the insertion of depth electrodes in her right temporal lobe showed that indeed her seizures had a localized focus there and that the surgical excision of that structure promised to ameliorate the disease significantly. The answer to the question of whether or not her right temporal lobe contributed to language called for the verification that her linguistic processing was rooted in the left hemisphere. This was indirectly calculated on the basis of her performance in a dichotic listening test, on the basis of her Oldfield handedness score, and on the basis of a new procedure conducted by Hanna Damasio, Peter Kirchner, and Karim Rezai in the Division of Nuclear Medicine of the Department of Radiology. Section A of the figure shows the resting state emission tomography study of the patient using Xenon-133 as radiotracer. It reveals a normal picture of resting activity with the usual activation of lateral cortices and basal ganglia as well as visual cortices in the occipital lobe caused by the fact that the subject was asked to have her eyes open during the procedure. But then the study was repeated in the following conditions: (a) the patient was told about a target word (e.g., the word able), before the study started and asked to detect any word that would rhyme with the target word while she listened to a series of more than 100 words given at five second intervals by means of an audio tape. When the patient detected one of the randomly inserted words that happened to rhyme with the target word, she signaled by a movement of the left foot. The patient did not have to produce any speech. This task calls not only for concentrated attention but also for intense phonemic processing as the patient holds the acoustical target in her mind and tries to spot the rhyming match, as it comes through, at unpredictable intervals.
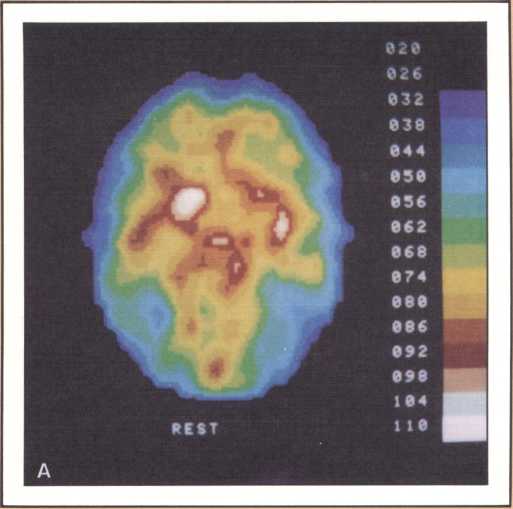
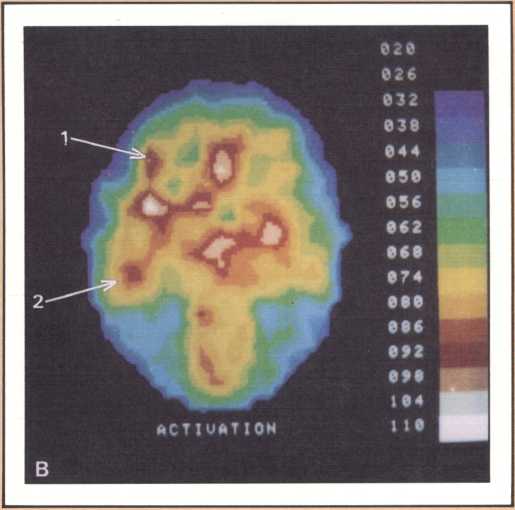
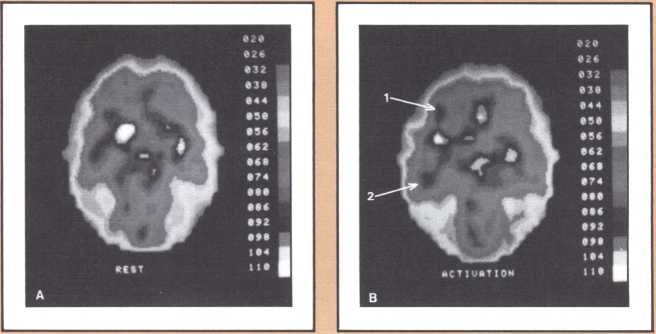
What happened during that second session of this noninvasive procedure (see figure 5B) was the selective activation of some areas in the patient’s brain, that had been quiet in her “resting” study. For instance, both an area that plotting revealed to be located in the left frontal lobe (Broca’s area) and an area corresponding to Wernicke’s area were activated. In addition, the basal ganglia and the right motor cortex, which guides the left foot area, were activated too, as a result of the patient’s repeated movements of the left foot to signal recognition of the rhyme. Finally, a large area of the left cerebellum, which was engaged in tandem with the right basal ganglia and right motor cortex in the performance of the movements of the left foot, showed increased activity.
This image should give us pause because it does highlight not only the power of modern technologies, but the simple fact, so often neglected, that the anatomical organization of the brain is the key to its action, that most functions occur in specified areas, that are part of a network, and unfortunately are not likely to occur anywhere else, hence the real drama of the loss of brain tissue in neurological disease. To get back to our subject, this probe gave us a final and stronger indication that indeed the patient’s English language was rooted in the left hemisphere. To be sure of this in yet one more way, Van Gilder, Lindell Gentry, and ourselves, also conducted a Wada test, a standard and still mandatory procedure in such cases, that consists of the introduction of a barbiturate into one side of the brain circulation, for just a brief period, in such a way that most brain activity in that particular area is temporarily suspended, as a consequence of the pharmacological action of the drug. During injection of sodium-amytal into the left hemisphere, we verified that the patient became transiently but severely aphasic, thus confirming the dedication of her left brain structures to aphasia. The epilogue of this story is a fortunate one. Van Gilder did carry out the surgery, and not only has the patient not had any other seizures since, but her language and other higher behaviors and cognition have been entirely unchanged. As you can see, these procedures were required to give physicians a handle on how to proceed therapeutically, with as little risk as possible for the patient. But just consider the amount of information that appropriate planning of the tasks permitted us to gather in the course of diagnostic management, and the benefit that both this patient and future patients harvested from this fresh knowledge.
The same activation task has been used, in patients in whom right hemisphere language was suspected. Such patients have showed increased activity of the right Broca’s and Wernicke’s areas.
Brain and Vision
Another type of striking behavioral dissociation was noted in the patient whose scan appears in figure 6. When a vascular lesion destroyed an area of the internal aspect of the occipital lobe in the left side, he suddenly became unable to read. He could see letters and words but was no longer able to evoke their sounds or decipher their meaning. Yet, he was able to write, and speak, and understand speech. He had the syndrome of alexia (impairment of reading) without agraphia (impairment of writing). More than 30 such subjects have been studied anatomically and behaviorally in our laboratory and compared with anatomical and behavioral controls. We came to the conclusion that the causative lesion had to be located in a very narrow area of cerebral tissue in order for alexia to appear in this relatively pure form. Incidentally, when this lesion is found closer to the pole of the occipital lobe, it produces another striking syndrome, a pure disorder of color perception in the field of vision contralateral to the side of the damage (figure 7). On the contrary, when the lesion is located higher, in the direction of the calcarine cortex (the primary visual cortex), it produces an impairment of form perception in the opposite visual field. The term for that is hemianopia, that is, a blind half field of vision (figure 8).
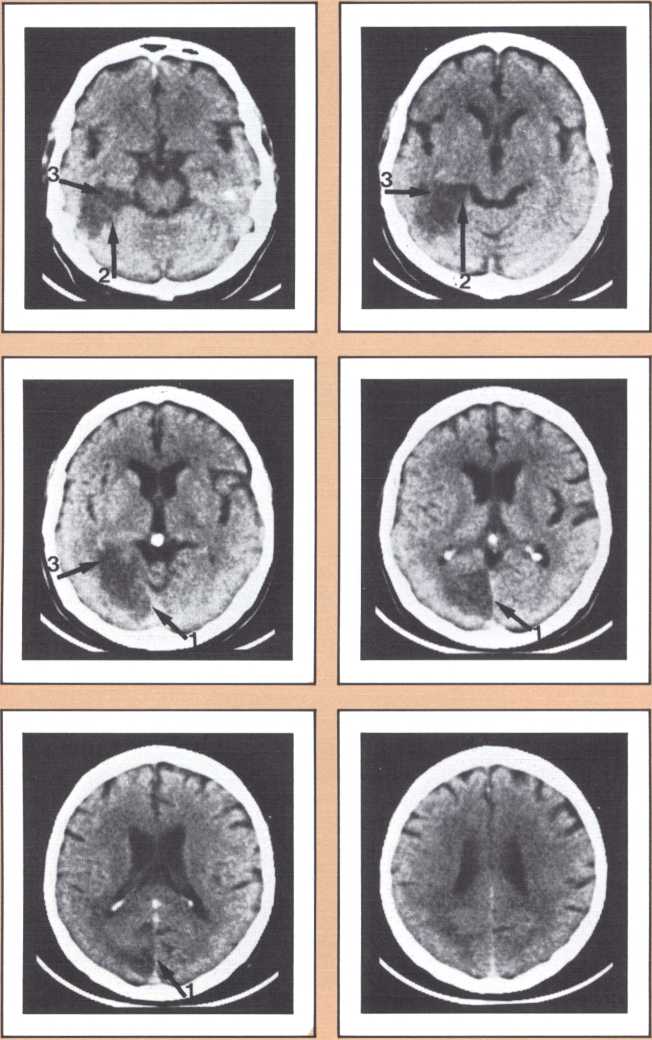
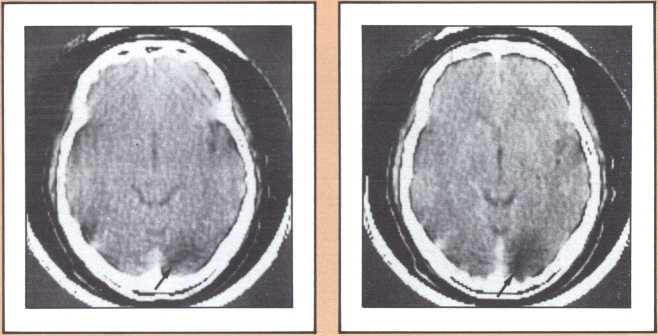
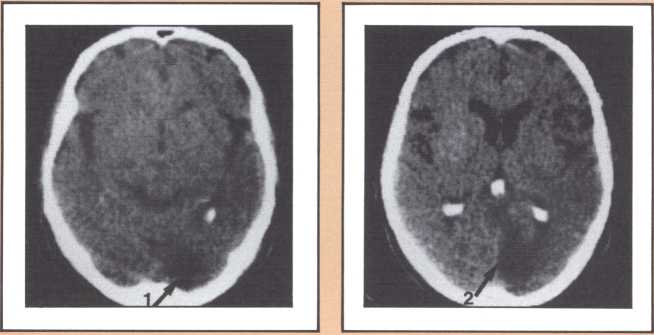
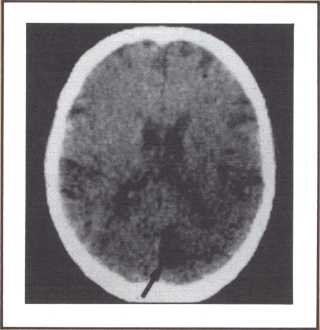
When this lesion is doubled and both sides of the occipital lobe are damaged, one of the most staggering disorders seen in human pathology may ensue: a selective impairment of facial recognition known as prosopagnosia (figure 9). Patients with prosopagnosia become unable to recognize faces previously known to them. This includes their own faces when they look at themselves in the mirror. In other words, a well-perceived familiar face does not trigger the pertinent associated memories that to any of us in this room will permit the conjuring up of the identity of the possessor of that face. No experience of familiarity takes place. Yet, such patients are immediately able to recognize the owner of the face by simply listening to the voice. Prosopagnosia is thus a visually triggered impairment of memory that clearly occurs beyond what one could call, for purposes of explanation, basic perception, and before the complex operation of memory evocation on which recognition is based.
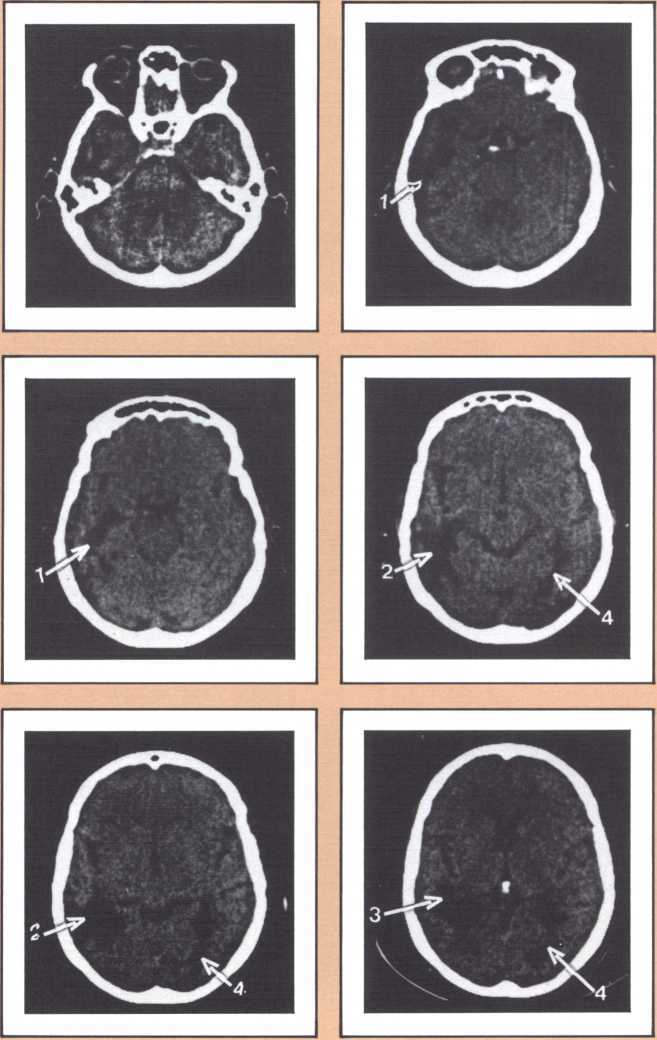
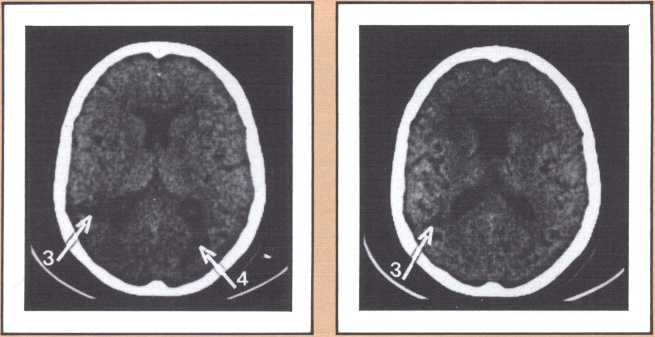
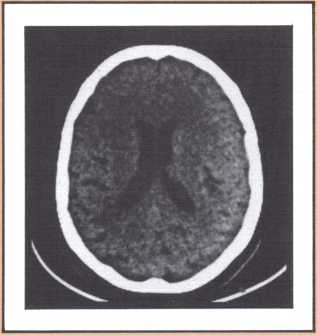
Experimental analysis of this disorder affords one of the best windows on the workings of human recall, recognition, and learning. The probing of what goes on, physiologically, when such lesions occur and patients become prosopagnosic is a fascinating task. In the model we have developed to explain both normal facial recognition and its impairment, we have postulated the existence of several physiological steps. In the first, the ongoing percept of a face is matched to a unique specific template of that face (the template system for each face depends on medial visual association cortices). The second is an activation step, during which the matched template calls up, by means of structures in the anterior temporal cortices, a variety of associated memories stored in diverse points of the modality-specific association cortices. The demonstration that this is indeed so, hinged on the proof that indeed there was template matching in the visual cortex. Daniel Tranel, a neuropsychologist in my laboratory, has developed such a probe using psychophysiological skin conductance responses (SCRs). In a preliminary study, he demonstrated that human faces, and especially familiar human faces, have high “signal value,” that is, normal human beings have significant SCRs to those faces. Then, in a variety of experiments in prosopagnosic subjects, Tranel and I demonstrated that the presentation of familiar faces which the subject is entirely unable to recognize visually, produced consistent skin conductance responses of high magnitude. On the contrary, the unfamiliar faces within which the familiar faces were randomly interspersed did not produce such responses. The difference between familiar faces (target faces) and unfamiliar faces (nontarget) was highly significant, overall and instance by instance. Clearly, these subjects are responding, at the autonomic level of their nervous system, to these faces. That means, unequivocally, that some part of their brains is recognizing these faces which they consciously are not able to know. Neither the knowledge that permits the “autonomic” recognition, nor the effects of that recognition ever reach awareness, and the subject’s behavior does not appear influenced at all by the positive recognition that is being carried out. We are thus witnessing an example of recognition without awareness, but we also believe that, at the same time, we are witnessing proof for the existence of visual templates for the specific faces (see Tranel & Damasio, Science, 1985).
I regret I do not have the time to tell you about other exciting investigations in this area now under way in our laboratories. Matthew Rizzo and Richard Hurtig, helped by James Corbett and H. Stanley Thompson, are studying the psychophysic profile and scan path performance of prosopagnosic patients and showing that their visual perception and visual exploratory strategies are largely normal.
Brain and Memory
In the 1950s, Milner and Scoville discovered that the surgical ablation of the medial, or internal, part of both temporal lobes caused a major form of memory impairment pertaining to material processed in any sensory modality or in any combination of modalities. The impairment effectively precluded learning in the forward direction, that is, learning of stimuli or events experienced after the surgical ablation of the medial areas of the temporal lobe. Analysis of the inaugural case, patient HM, has revealed an intriguing set of associations regarding memory and learning. First of all, HM has been unable to learn, consciously, practically any new material of the type that a person of his age, intelligence, and education would master in no time. But there is one major exception: HM can learn visuomotor skills at a rate comparable to controls, thus indicating that this form of amnesia does spare motor learning. Furthermore, when you consider the experiments I have just reported on facial recognition, it must be apparent that the statement that HM is entirely unable to learn has to be qualified. He is unable to learn in such a way that knowledge can be brought into consciousness and used in willful behavior, and so he cannot recall or recognize with awareness. But it is perhaps not true that he is entirely unable to learn. Another major dissociation has also become apparent: HM’s retrograde memory, that is, the wealth of knowledge he acquired prior to the development of his brain lesions, is intact. There is a split between his impaired anterograde memory and his intact retrograde memory.
It was against this background that we were able to find, now almost nine years ago, the first of a series of subjects that was radically different from HM in this latter and major feature. He is known by the initials DRB and his retrograde memory was entirely demolished as far as contextual knowledge was concerned, that is, he was not able to recall any event or episode that happened in his past life; he was unable to recognize any of the previously familiar faces he was acquainted with; he was unable to place any visual, or auditory, verbal, or nonverbal bit of information in its proper, personal, historical perspective. Yet, his knowledge of the universe, in generic terms, was entirely preserved. (The term generic (or semantic) memory denotes knowledge about the basic properties, such as class membership and function. Contextual (or episodic) memory denotes knowledge of a specific stimulus, its relationship to other stimuli in a given situation, its relationship to the perceiver, and may contain a temporal element. The concepts of episodic and semantic memory were proposed by Tulving (1972). The terms contextual and generic are our own.) In a variety of experiments we ascertained that this person still maintained isolated components of complex memories, unhinged, available in erratic, unbound manner. The shreds of memory he was able to conjure up, on occasion, never helped him evoke the whole event they belonged to. Formally, his recall and recognition of contextual memory were zero.
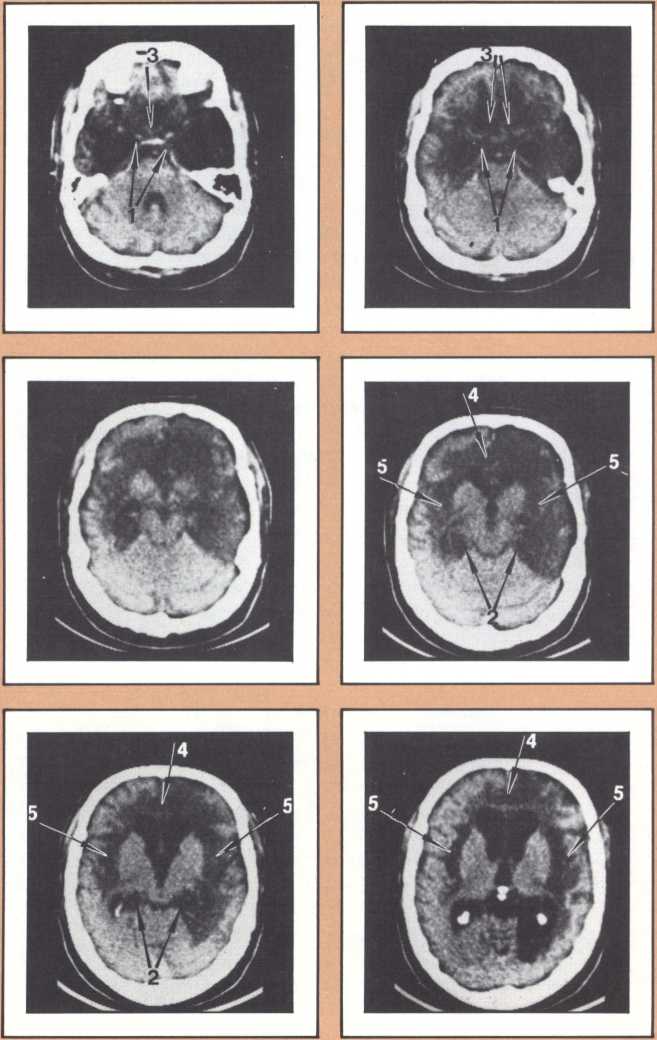
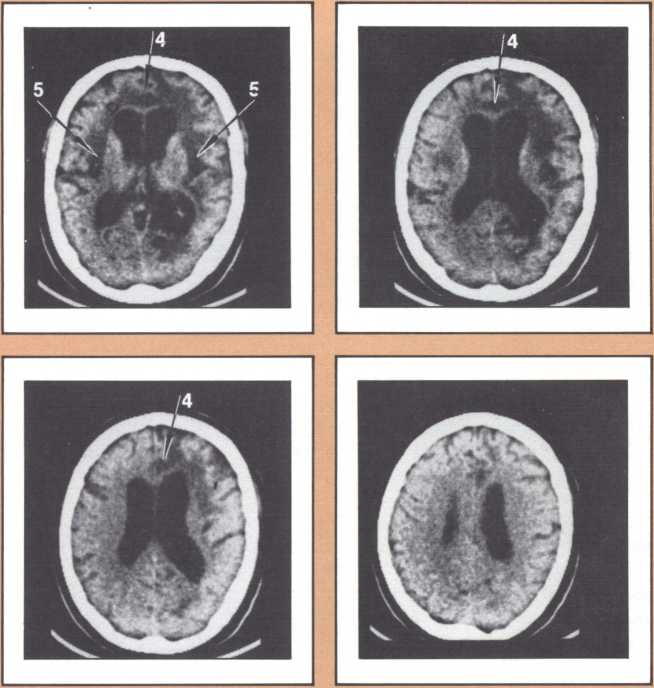
The neuroimaging study of this patient shows, without a doubt, that both the medial temporal lobe region bilaterally and the lateral and polar temporal lobe regions bilaterally, were destroyed. In patient HM, on the contrary, only the medial temporal region was destroyed. In short, we believe that while patient HM has suffered the bilateral loss of the hippocampal formation and the adjoining entorhinal cortex and amygdala, patient DRB has lost not only those structures but also cortical units in the pole in the lateral aspects of the temporal lobe (figure 10). We believe those additional lesions are critically responsible for the pervasive retrograde memory loss that patient DRB shows but not patient HM. These findings led to the formulation of a hypothesis we are currently investigating: (a) that structures in the medial aspect of the temporal lobe, such as the hippocampus, the entorhinal cortex, and the subiculum, are necessary to computate the co-occurrence of processing of different stimuli and thus for the formation of a multientry, multiindex catalog; (b) that this abstract catalog is probably supported, structurally, by neural units in the cortices of the pole and lateral aspect of the temporal lobe, as well as lateral frontal lobe.
More recently, we have also been able to study yet another form of amnesic syndrome. The condition is milder. One may describe it as a partial amnesia. Both the making of new memory and the recall or recognition of old memories are disturbed. The temporal co-registering of the separate components of new experiences appears more impaired. The damage associated with such amnesic syndromes is structurally located in an area of the brain that one decade ago few neurologists would even think about in connection to either disease or normal function. That is the basal forebrain, a gray matter region located underneath and in front of the basal ganglia, close to the midline, in a geographic no-man’s-land between the frontal lobe and the deep gray matter of the brain. The area includes the septal nuclei, the nucleus accumbens, the substantia innominata, the last of which contains in part the nucleus basalis of Meynert, one of the principal providers of the neurochemical mediator acetylcholine to widespread areas of the brain. The story that will unfold from these studies will be an interesting one. Already we know that although the damage is largely confined to this small area, there are additional areas of dysfunction in the temporal regions of the brain, especially in the lateral regions where we believe the physical support of the catalog exists, and also in the medial structures of the thalamus in a region that other types of amnesic syndromes have indicated is also related to memory.
Memory Impairment and Alzheimer’s Disease
The subject of memory brings us to our closing topic, Alzheimer’s disease. It was because of our interest in memory that we became interested in the underpinnings of this disorder, which as you all know is characterized by a gradual, relentless loss of memory. The loss, very early on, is of the type I first described for patient HM, an impairment of the acquisition or recall of contextual memories. The knowledge accumulated in the other amnesic patients in combination with detailed information on the anatomical structure of the hippocampus that also surfaced within the last decade (in great part due to the outstanding studies of Gary Van Hoesen in the rhesus monkey) led Van Hoesen and myself, with the help of our postdoctoral student, Bradley Hyman, to investigate the anatomical placement of the characteristic lesions of Alzheimer’s disease within a set of structures that, our hypothesis predicted, were likely not to operate normally in this disease. Again, some years elapsed during which we studied some of the victims of this tragic illness and approached, as reasonably and humanely as possible, the most perceptive relatives to the great potential benefit for detailed post mortem analysis of the patients’ brains. These efforts have been rewarded by some striking findings.
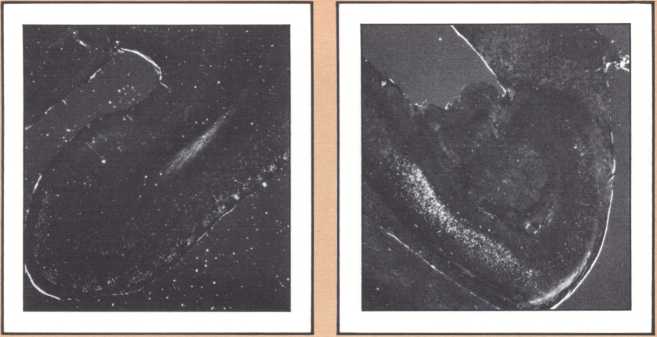
The studies revealed a clear pattern of damage within brain structures known to be associated with memory processing, while no such changes were seen in the brains of age-matched controls without Alzheimer’s disease. Within the temporal lobe, the major concentration of diseased cells, as noted by the presence of neurofibrillary tangles, was in the entorhinal cortex and in the subiculum (figure 11 A & B). The finding is especially intriguing considering the role of these structures. The entorhinal cortex is the portion of temporal lobe cortex that serves as the major staging area for information flow into the hippocampus. In other words, sensory information coming from auditory, visual, or somatosensory centers in the brain can only enter the hippocampus by way of the entorhinal cortex. It does so by means of cellular connections in the second layer off that cortex, and the cells of that layer were massively destroyed in the brains of our patients. The subiculum, on the other hand, constitutes the staging area for outflow from the hippocampus. After information has been processed by the hippocampus, the results are communicated to both cortical and subcortical structures via pathways that originate in the subiculum. Again, damaged cells were massively concentrated here. Curiously, the area which receives, processes, and sends back processed information, the hippocampal formation itself, was largely intact as far as the presence of neurofibrillary tangles was concerned. The result of this anatomical distribution of pathology was that the hippocampus of these Alzheimer’s patients was virtually isolated from the remainder of the cerebral cortex. The damage at both the input and output conduit areas disconnected the hippocampus and thus rendered inoperative a structure crucial to the acquisition of new memories.
It is reasonable to hypothesize that the early defects of memory in patients with Alzheimer’s disease are the result of these specific forms of damage. But not all forms of memory impairment in Alzheimer’s are the consequence of these lesions. As the disease progresses other types of memory change develop, and we believe other mechanisms must account for them. Studies currently being conducted in our laboratories, using fine neuroimaging techniques, will shed some light on those other aspects of memory loss (figure 12).
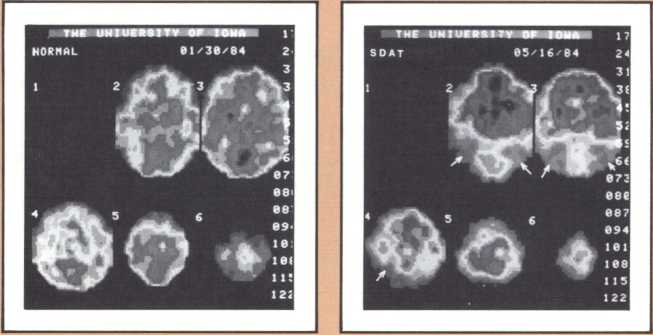
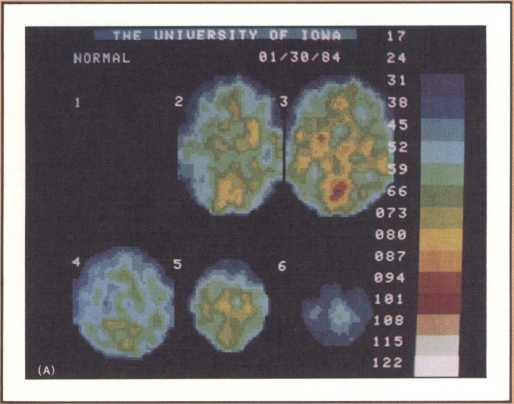
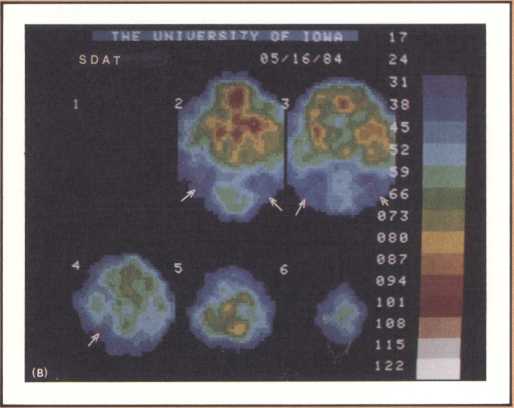
The most surprising finding in our study was the selectivity with which the disease dissects one particular type of cortex and even one particular cell layer, leaving intact neighboring parts of the cortex and other cell groups. What findings such as these may signify is that the cause, or causes, of Alzheimer’s are of such a nature that, at least in the early phase of the disease, they attack certain cell groups within given brain regions, while leaving others intact. The inevitable question, then, is why these specific cell groups are more vulnerable than their neighbors to the disease process, or processes, that cause Alzheimer’s. These are unanswered questions but they are clues for further investigation.
Closing Statements
I have presented a few samples of areas of investigation where some exciting results are being obtained and then only with material from our own work at the College of Medicine. The message is that we are now able to harness some neurological diseases and make them contribute to our understanding of brain function in a humane, thoroughly ethical, fruitful scientific setting; and that, conversely, the results of such science are not only advancing fundamental knowledge but permitting the development of diagnostic, therapeutic, and preventive measures that are likely to diminish or even eradicate disease. The ability to expand our comprehension of mechanisms of disease and of the relation between brain and mind, inasmuch as it depends on the neurosciences, is now limited only by frontiers of technology, imagination, and intelligence. But comprehension of the full scope of human higher behavior does not depend on neuroscience alone. The ultimate meaning of the brain/mind relationships we now glimpse will depend on the bridges that neuroscience establishes with the nonmedical domains of the humanities-history, sociology, anthropology, philosophy, psychology, and linguistics, the world of the creative artist, to name but the most visible partners. That is a fundamental reason why we need, perhaps more than ever, the humanly rich, the intellectually and physically integrated environment of a university.
Understanding normal function and disease is a different matter from conquering brain disease. The limits to that potential conquest are not only human imagination and technology. They are also the blueprint of man’s physical constitution, the well-weighed endowment that each of us carries as a result of genetic inheritance and environmental interaction since we started to evolve in utero. It will become technically feasible to intervene in both the genetic programming and in the environment of a fetus but the decision as to whether we should, or when we should, will confront us, much like a sorcerer’s apprentice. It will be even possible to perform brain transplants from and to cerebral servosystems, those lower systems that are less rigid functionally.
In the meantime, the future is indeed promising, especially if society, in its many facets, realizes that resources for scientific endeavors must not dwindle, that scientific success depends on the availability of an increasing pool of imaginative and well-trained scientists.
The picture that I would like to leave in your minds is that of my firm belief, that no single approach is likely to solve, by itself, any of the major problems of brain function and disease but that a concerted effort will. From our vantage point, I have shown you a few components of the human brain that modern ink may character, and told you how, in the proper setting, they contribute to our understanding of how we are what we are.